An anatomically comprehensive atlas of the adult human brain transcriptome
- PMID: 22996553
- PMCID: PMC4243026
- DOI: 10.1038/nature11405
An anatomically comprehensive atlas of the adult human brain transcriptome
Abstract
Neuroanatomically precise, genome-wide maps of transcript distributions are critical resources to complement genomic sequence data and to correlate functional and genetic brain architecture. Here we describe the generation and analysis of a transcriptional atlas of the adult human brain, comprising extensive histological analysis and comprehensive microarray profiling of ∼900 neuroanatomically precise subdivisions in two individuals. Transcriptional regulation varies enormously by anatomical location, with different regions and their constituent cell types displaying robust molecular signatures that are highly conserved between individuals. Analysis of differential gene expression and gene co-expression relationships demonstrates that brain-wide variation strongly reflects the distributions of major cell classes such as neurons, oligodendrocytes, astrocytes and microglia. Local neighbourhood relationships between fine anatomical subdivisions are associated with discrete neuronal subtypes and genes involved with synaptic transmission. The neocortex displays a relatively homogeneous transcriptional pattern, but with distinct features associated selectively with primary sensorimotor cortices and with enriched frontal lobe expression. Notably, the spatial topography of the neocortex is strongly reflected in its molecular topography-the closer two cortical regions, the more similar their transcriptomes. This freely accessible online data resource forms a high-resolution transcriptional baseline for neurogenetic studies of normal and abnormal human brain function.
Figures
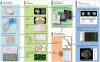
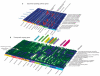
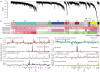
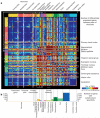
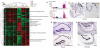
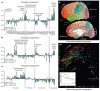
Comment in
-
Neurogenetics: mapping gene expression in the human brain.Nat Rev Neurosci. 2012 Nov;13(11):737. doi: 10.1038/nrn3370. Epub 2012 Oct 17. Nat Rev Neurosci. 2012. PMID: 23072913 No abstract available.
-
The genetic essence of our brains.Nat Methods. 2012 Nov;9(11):1044-5. doi: 10.1038/nmeth.2236. Nat Methods. 2012. PMID: 23281566 No abstract available.
Similar articles
-
Transcriptional landscape of the prenatal human brain.Nature. 2014 Apr 10;508(7495):199-206. doi: 10.1038/nature13185. Epub 2014 Apr 2. Nature. 2014. PMID: 24695229 Free PMC article.
-
Transcriptional architecture of the primate neocortex.Neuron. 2012 Mar 22;73(6):1083-99. doi: 10.1016/j.neuron.2012.03.002. Epub 2012 Mar 21. Neuron. 2012. PMID: 22445337 Free PMC article.
-
The Allen Human Brain Atlas: comprehensive gene expression mapping of the human brain.Trends Neurosci. 2012 Dec;35(12):711-4. doi: 10.1016/j.tins.2012.09.005. Epub 2012 Oct 5. Trends Neurosci. 2012. PMID: 23041053
-
Cellular distribution of the calcium-binding proteins parvalbumin, calbindin, and calretinin in the neocortex of mammals: phylogenetic and developmental patterns.J Chem Neuroanat. 1999 Feb;16(2):77-116. doi: 10.1016/s0891-0618(98)00065-9. J Chem Neuroanat. 1999. PMID: 10223310 Review.
-
Brain transcriptome atlases: a computational perspective.Brain Struct Funct. 2017 May;222(4):1557-1580. doi: 10.1007/s00429-016-1338-2. Epub 2016 Dec 1. Brain Struct Funct. 2017. PMID: 27909802 Free PMC article. Review.
Cited by
-
Induction of a common microglia gene expression signature by aging and neurodegenerative conditions: a co-expression meta-analysis.Acta Neuropathol Commun. 2015 May 23;3:31. doi: 10.1186/s40478-015-0203-5. Acta Neuropathol Commun. 2015. PMID: 26001565 Free PMC article.
-
Neurogenetics: mapping gene expression in the human brain.Nat Rev Neurosci. 2012 Nov;13(11):737. doi: 10.1038/nrn3370. Epub 2012 Oct 17. Nat Rev Neurosci. 2012. PMID: 23072913 No abstract available.
-
Ultrafast and interpretable single-cell 3D genome analysis with Fast-Higashi.Cell Syst. 2022 Oct 19;13(10):798-807.e6. doi: 10.1016/j.cels.2022.09.004. Cell Syst. 2022. PMID: 36265466 Free PMC article.
-
Allen Brain Atlas: an integrated spatio-temporal portal for exploring the central nervous system.Nucleic Acids Res. 2013 Jan;41(Database issue):D996-D1008. doi: 10.1093/nar/gks1042. Epub 2012 Nov 28. Nucleic Acids Res. 2013. PMID: 23193282 Free PMC article.
-
Shaping brain structure: Genetic and phylogenetic axes of macroscale organization of cortical thickness.Sci Adv. 2020 Sep 25;6(39):eabb3417. doi: 10.1126/sciadv.abb3417. Print 2020 Sep. Sci Adv. 2020. PMID: 32978162 Free PMC article.
References
Publication types
MeSH terms
Substances
Grants and funding
LinkOut - more resources
Full Text Sources
Other Literature Sources
Molecular Biology Databases