A kinetic clutch governs religation by type IB topoisomerases and determines camptothecin sensitivity
- PMID: 22991469
- PMCID: PMC3479559
- DOI: 10.1073/pnas.1206480109
A kinetic clutch governs religation by type IB topoisomerases and determines camptothecin sensitivity
Abstract
Type IB topoisomerases (Top1Bs) relax excessive DNA supercoiling associated with replication and transcription by catalyzing a transient nick in one strand to permit controlled rotation of the DNA about the intact strand. The natural compound camptothecin (CPT) and the cancer chemotherapeutics derived from it, irinotecan and topotecan, are highly specific inhibitors of human nuclear Top1B (nTop1). Previous work on vaccinia Top1B led to an elegant model that describes a straightforward dependence of rotation and religation on the torque caused by supercoiling. Here, we used a single-molecule DNA supercoil relaxation assay to measure the torque dependence of nTop1 and its inhibition by CPT. For comparison, we also examined mitochondrial Top1B and an N-terminal deletion mutant of nTop1. Despite substantial sequence homology in their core domains, nTop1 and mitochondrial Top1B exhibit dramatic differences in sensitivity to torque and CPT, with the N-terminal deletion mutant of nTop1 showing intermediate characteristics. In particular, nTop1 displays nearly torque-independent religation probability, distinguishing it from other Top1B enzymes studied to date. Kinetic modeling reveals a hitherto unobserved torque-independent transition linking the DNA rotation and religation phases of the enzymatic cycle. The parameters of this transition determine the torque sensitivity of religation and the efficiency of CPT binding. This "kinetic clutch" mechanism explains the molecular basis of CPT sensitivity and more generally provides a framework with which to interpret Top1B activity and inhibition.
Conflict of interest statement
The authors declare no conflict of interest.
Figures
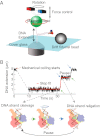
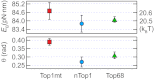

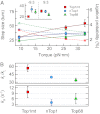


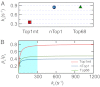
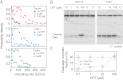
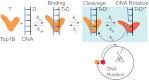
Similar articles
-
Vaccinia DNA topoisomerase I: evidence supporting a free rotation mechanism for DNA supercoil relaxation.Biochemistry. 1997 Apr 29;36(17):5212-22. doi: 10.1021/bi962880t. Biochemistry. 1997. PMID: 9136883
-
Structural insights into the function of type IB topoisomerases.Curr Opin Struct Biol. 1999 Feb;9(1):29-36. doi: 10.1016/s0959-440x(99)80005-0. Curr Opin Struct Biol. 1999. PMID: 10047584
-
Single-Molecule Supercoil Relaxation Assay as a Screening Tool to Determine the Mechanism and Efficacy of Human Topoisomerase IB Inhibitors.Mol Cancer Ther. 2015 Nov;14(11):2552-9. doi: 10.1158/1535-7163.MCT-15-0454. Epub 2015 Sep 8. Mol Cancer Ther. 2015. PMID: 26351326 Free PMC article.
-
[DNA supercoiling and topoisomerases in Escherichia coli].Rev Latinoam Microbiol. 1995 Jul-Sep;37(3):291-304. Rev Latinoam Microbiol. 1995. PMID: 8850348 Review. Spanish.
-
Role of flexibility and long range communication on the function of human topoisomerase I.Ital J Biochem. 2007 Jun;56(2):110-4. Ital J Biochem. 2007. PMID: 17722651 Review.
Cited by
-
TOP1 CAD-seq: A protocol to map catalytically engaged topoisomerase 1 in human cells.STAR Protoc. 2022 Aug 1;3(3):101581. doi: 10.1016/j.xpro.2022.101581. eCollection 2022 Sep 16. STAR Protoc. 2022. PMID: 35942340 Free PMC article.
-
Gold(III) macrocycles: nucleotide-specific unconventional catalytic inhibitors of human topoisomerase I.J Am Chem Soc. 2014 Apr 16;136(15):5670-82. doi: 10.1021/ja412350f. Epub 2014 Apr 2. J Am Chem Soc. 2014. PMID: 24694294 Free PMC article.
-
Synthesis and biological evaluation of new carbohydrate-substituted indenoisoquinoline topoisomerase I inhibitors and improved syntheses of the experimental anticancer agents indotecan (LMP400) and indimitecan (LMP776).J Med Chem. 2014 Feb 27;57(4):1495-512. doi: 10.1021/jm401814y. Epub 2014 Feb 11. J Med Chem. 2014. PMID: 24517248 Free PMC article.
-
Simulation of DNA Supercoil Relaxation.Biophys J. 2016 May 24;110(10):2176-84. doi: 10.1016/j.bpj.2016.03.041. Biophys J. 2016. PMID: 27224483 Free PMC article.
-
Torsionally constrained DNA for single-molecule assays: an efficient, ligation-free method.Nucleic Acids Res. 2013 Oct;41(19):e179. doi: 10.1093/nar/gkt699. Epub 2013 Aug 9. Nucleic Acids Res. 2013. PMID: 23935118 Free PMC article.
References
Publication types
MeSH terms
Substances
Grants and funding
LinkOut - more resources
Full Text Sources
Research Materials