Comparative dynamics of NMDA- and AMPA-glutamate receptor N-terminal domains
- PMID: 22959625
- PMCID: PMC3496038
- DOI: 10.1016/j.str.2012.08.012
Comparative dynamics of NMDA- and AMPA-glutamate receptor N-terminal domains
Abstract
Ionotropic glutamate receptors (iGluRs) harbor two extracellular domains: the membrane-proximal ligand-binding domain (LBD) and the distal N-terminal domain (NTD). These are involved in signal sensing: the LBD binds L-glutamate, which activates the receptor channel. Ligand binding to the NTD modulates channel function in the NMDA receptor subfamily of iGluRs, which has not been observed for the AMPAR subfamily to date. Structural data suggest that AMPAR NTDs are packed into tight dimers and have lost their signaling potential. Here, we assess NTD dynamics from both subfamilies, using a variety of computational tools. We describe the conformational motions that underly NMDAR NTD allosteric signaling. Unexpectedly, AMPAR NTDs are capable of undergoing similar dynamics; although dimerization imposes restrictions, the two subfamilies sample similar, interconvertible conformational subspaces. Finally, we solve the crystal structure of AMPAR GluA4 NTD, and combined with molecular dynamics simulations, we characterize regions pivotal for an as-yet-unexplored dynamic spectrum of AMPAR NTDs.
Copyright © 2012 Elsevier Ltd. All rights reserved.
Figures
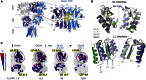
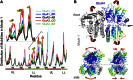
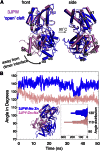
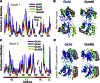
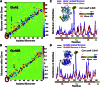
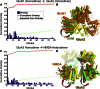
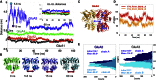
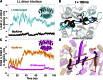
Similar articles
-
Structure, Dynamics, and Allosteric Potential of Ionotropic Glutamate Receptor N-Terminal Domains.Biophys J. 2015 Sep 15;109(6):1136-48. doi: 10.1016/j.bpj.2015.06.061. Epub 2015 Aug 6. Biophys J. 2015. PMID: 26255587 Free PMC article. Review.
-
Cooperative Dynamics of Intact AMPA and NMDA Glutamate Receptors: Similarities and Subfamily-Specific Differences.Structure. 2015 Sep 1;23(9):1692-1704. doi: 10.1016/j.str.2015.07.002. Epub 2015 Aug 6. Structure. 2015. PMID: 26256538 Free PMC article.
-
Druggability Simulations and X-Ray Crystallography Reveal a Ligand-Binding Site in the GluA3 AMPA Receptor N-Terminal Domain.Structure. 2019 Feb 5;27(2):241-252.e3. doi: 10.1016/j.str.2018.10.017. Epub 2018 Dec 6. Structure. 2019. PMID: 30528594 Free PMC article.
-
Dynamics and allosteric potential of the AMPA receptor N-terminal domain.EMBO J. 2011 Mar 2;30(5):972-82. doi: 10.1038/emboj.2011.17. Epub 2011 Feb 11. EMBO J. 2011. PMID: 21317871 Free PMC article.
-
Activation and desensitization of ionotropic glutamate receptors by selectively triggering pre-existing motions.Neurosci Lett. 2019 May 1;700:22-29. doi: 10.1016/j.neulet.2018.02.050. Epub 2018 Feb 23. Neurosci Lett. 2019. PMID: 29481851 Free PMC article. Review.
Cited by
-
Multi-scale modeling of the circadian modulation of learning and memory.PLoS One. 2019 Jul 19;14(7):e0219915. doi: 10.1371/journal.pone.0219915. eCollection 2019. PLoS One. 2019. PMID: 31323054 Free PMC article.
-
The N-terminal domain modulates α-amino-3-hydroxy-5-methyl-4-isoxazolepropionic acid (AMPA) receptor desensitization.J Biol Chem. 2014 May 9;289(19):13197-205. doi: 10.1074/jbc.M113.526301. Epub 2014 Mar 20. J Biol Chem. 2014. PMID: 24652293 Free PMC article.
-
Probing the Structural Dynamics of the NMDA Receptor Activation by Coarse-Grained Modeling.Biophys J. 2017 Jun 20;112(12):2589-2601. doi: 10.1016/j.bpj.2017.04.043. Biophys J. 2017. PMID: 28636915 Free PMC article.
-
Structure, Dynamics, and Allosteric Potential of Ionotropic Glutamate Receptor N-Terminal Domains.Biophys J. 2015 Sep 15;109(6):1136-48. doi: 10.1016/j.bpj.2015.06.061. Epub 2015 Aug 6. Biophys J. 2015. PMID: 26255587 Free PMC article. Review.
-
An inter-dimer allosteric switch controls NMDA receptor activity.EMBO J. 2019 Jan 15;38(2):e99894. doi: 10.15252/embj.201899894. Epub 2018 Nov 5. EMBO J. 2019. PMID: 30396997 Free PMC article.
References
-
- Armstrong N., Gouaux E. Mechanisms for activation and antagonism of an AMPA-sensitive glutamate receptor: crystal structures of the GluR2 ligand binding core. Neuron. 2000;28:165–181. - PubMed
-
- Ayalon G., Stern-Bach Y. Functional assembly of AMPA and kainate receptors is mediated by several discrete protein-protein interactions. Neuron. 2001;31:103–113. - PubMed
-
- Bahadur R.P., Chakrabarti P., Rodier F., Janin J. A dissection of specific and non-specific protein-protein interfaces. J. Mol. Biol. 2004;336:943–955. - PubMed
Publication types
MeSH terms
Substances
Grants and funding
LinkOut - more resources
Full Text Sources
Molecular Biology Databases