Anaesthesia and physiological monitoring during in vivo imaging of laboratory rodents: considerations on experimental outcomes and animal welfare
- PMID: 22877315
- PMCID: PMC3467189
- DOI: 10.1186/2191-219X-2-44
Anaesthesia and physiological monitoring during in vivo imaging of laboratory rodents: considerations on experimental outcomes and animal welfare
Abstract
The implementation of imaging technologies has dramatically increased the efficiency of preclinical studies, enabling a powerful, non-invasive and clinically translatable way for monitoring disease progression in real time and testing new therapies. The ability to image live animals is one of the most important advantages of these technologies. However, this also represents an important challenge as, in contrast to human studies, imaging of animals generally requires anaesthesia to restrain the animals and their gross motion. Anaesthetic agents have a profound effect on the physiology of the animal and may thereby confound the image data acquired. It is therefore necessary to select the appropriate anaesthetic regime and to implement suitable systems for monitoring anaesthetised animals during image acquisition. In addition, repeated anaesthesia required for longitudinal studies, the exposure of ionising radiations and the use of contrast agents and/or imaging biomarkers may also have consequences on the physiology of the animal and its response to anaesthesia, which need to be considered while monitoring the animals during imaging studies. We will review the anaesthesia protocols and monitoring systems commonly used during imaging of laboratory rodents. A variety of imaging modalities are used for imaging rodents, including magnetic resonance imaging, computed tomography, positron emission tomography, single photon emission computed tomography, high frequency ultrasound and optical imaging techniques such as bioluminescence and fluorescence imaging. While all these modalities are implemented for non-invasive in vivo imaging, there are certain differences in terms of animal handling and preparation, how the monitoring systems are implemented and, importantly, how the imaging procedures themselves can affect mammalian physiology. The most important and critical adverse effects of anaesthetic agents are depression of respiration, cardiovascular system disruption and thermoregulation. When anaesthetising rodents, one must carefully consider if these adverse effects occur at the therapeutic dose required for anaesthesia, if they are likely to affect the image acquisitions and, importantly, if they compromise the well-being of the animals. We will review how these challenges can be successfully addressed through an appropriate understanding of anaesthetic protocols and the implementation of adequate physiological monitoring systems.
Figures
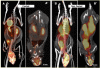
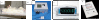
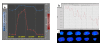
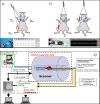
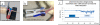
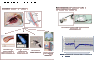
Similar articles
-
Imaging technologies and basic considerations for welfare of laboratory rodents.Lab Anim (NY). 2015 Mar;44(3):97-105. doi: 10.1038/laban.665. Lab Anim (NY). 2015. PMID: 25693107 Review.
-
Imaging technologies for preclinical models of bone and joint disorders.EJNMMI Res. 2011 Jul 29;1(1):11. doi: 10.1186/2191-219X-1-11. EJNMMI Res. 2011. PMID: 22214535 Free PMC article.
-
[Laboratory animal anaesthesia: influence of anaesthetic protocols on experimental models].Ann Fr Anesth Reanim. 2004 Aug;23(8):811-8. doi: 10.1016/j.annfar.2004.05.013. Ann Fr Anesth Reanim. 2004. PMID: 15345253 Review. French.
-
Anesthesia and other considerations for in vivo imaging of small animals.ILAR J. 2008;49(1):17-26. doi: 10.1093/ilar.49.1.17. ILAR J. 2008. PMID: 18172330
-
Anaesthesia of farmed fish: implications for welfare.Fish Physiol Biochem. 2012 Feb;38(1):201-18. doi: 10.1007/s10695-011-9565-1. Epub 2011 Dec 9. Fish Physiol Biochem. 2012. PMID: 22160749 Review.
Cited by
-
Long-term stability of physiological signals within fluctuations of brain state under urethane anesthesia.PLoS One. 2021 Oct 25;16(10):e0258939. doi: 10.1371/journal.pone.0258939. eCollection 2021. PLoS One. 2021. PMID: 34695166 Free PMC article.
-
A longitudinal, multi-parametric functional MRI study to determine age-related changes in the rodent brain.Neuroimage. 2020 Sep;218:116976. doi: 10.1016/j.neuroimage.2020.116976. Epub 2020 May 25. Neuroimage. 2020. PMID: 32464290 Free PMC article.
-
A novel echocardiographic method closely agrees with cardiac magnetic resonance in the assessment of left ventricular function in infarcted mice.Sci Rep. 2019 Mar 5;9(1):3580. doi: 10.1038/s41598-019-40393-0. Sci Rep. 2019. PMID: 30837662 Free PMC article.
-
Intranasally administered IGF-1 inhibits spreading depression in vivo.Brain Res. 2017 Dec 15;1677:47-57. doi: 10.1016/j.brainres.2017.09.022. Epub 2017 Sep 23. Brain Res. 2017. PMID: 28951235 Free PMC article.
-
Recent Technical Advances in Accelerating the Clinical Translation of Small Animal Brain Imaging: Hybrid Imaging, Deep Learning, and Transcriptomics.Front Med (Lausanne). 2022 Mar 24;9:771982. doi: 10.3389/fmed.2022.771982. eCollection 2022. Front Med (Lausanne). 2022. PMID: 35402436 Free PMC article. Review.
References
LinkOut - more resources
Full Text Sources