The first five seconds in the life of a clathrin-coated pit
- PMID: 22863004
- PMCID: PMC3413093
- DOI: 10.1016/j.cell.2012.05.047
The first five seconds in the life of a clathrin-coated pit
Abstract
Coated pits assemble by growth of a clathrin lattice, which is linked by adaptors to the underlying membrane. How does this process start? We used live-cell TIRF imaging with single-molecule EGFP sensitivity and high temporal resolution to detect arrival of the clathrin triskelions and AP2 adaptors that initiate coat assembly. Unbiased object identification and trajectory tracking, together with a statistical model, yield the arrival times and numbers of individual proteins, as well as experimentally confirmed estimates of the extent of substitution of endogenous by expressed, fluorescently tagged proteins. Pits initiate by coordinated arrival of clathrin and AP2, which is usually detected as two sequential steps, each of one triskelion with two adaptors. PI-4,5-P2 is essential for initiation. The accessory proteins FCHo1/2 are not; instead, they are required for sustained growth. This objective picture of coated pit initiation also shows that methods outlined here will be broadly useful for studies of dynamic assemblies in living cells.
Copyright © 2012 Elsevier Inc. All rights reserved.
Figures
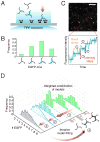
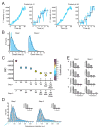
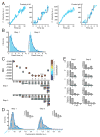
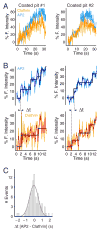
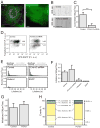
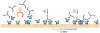
Comment in
-
How vesicles put on their coat.Nat Methods. 2012 Oct;9(10):948. doi: 10.1038/nmeth.2192. Nat Methods. 2012. PMID: 23193582 No abstract available.
Similar articles
-
Adaptor self-aggregation, adaptor-receptor recognition and binding of alpha-adaptin subunits to the plasma membrane contribute to recruitment of adaptor (AP2) components of clathrin-coated pits.EMBO J. 1993 May;12(5):2169-80. doi: 10.1002/j.1460-2075.1993.tb05865.x. EMBO J. 1993. PMID: 8491205 Free PMC article.
-
Endocytic clathrin-coated pit formation is independent of receptor internalization signal levels.Mol Biol Cell. 1998 May;9(5):1177-94. doi: 10.1091/mbc.9.5.1177. Mol Biol Cell. 1998. PMID: 9571248 Free PMC article.
-
Endocytosis of activated receptors and clathrin-coated pit formation: deciphering the chicken or egg relationship.J Cell Biol. 1996 Mar;132(6):1025-36. doi: 10.1083/jcb.132.6.1025. J Cell Biol. 1996. PMID: 8601582 Free PMC article.
-
Evolving models for assembling and shaping clathrin-coated pits.J Cell Biol. 2020 Sep 7;219(9):e202005126. doi: 10.1083/jcb.202005126. J Cell Biol. 2020. PMID: 32770195 Free PMC article. Review.
-
Adaptors for clathrin-mediated traffic.Annu Rev Cell Dev Biol. 1999;15:705-32. doi: 10.1146/annurev.cellbio.15.1.705. Annu Rev Cell Dev Biol. 1999. PMID: 10611976 Review.
Cited by
-
The Regulation of Exosome Generation and Function in Physiological and Pathological Processes.Int J Mol Sci. 2023 Dec 23;25(1):255. doi: 10.3390/ijms25010255. Int J Mol Sci. 2023. PMID: 38203424 Free PMC article. Review.
-
Imaging cell biology in live animals: ready for prime time.J Cell Biol. 2013 Jun 24;201(7):969-79. doi: 10.1083/jcb.201212130. J Cell Biol. 2013. PMID: 23798727 Free PMC article. Review.
-
The CHD Protein, Kismet, is Important for the Recycling of Synaptic Vesicles during Endocytosis.Sci Rep. 2019 Dec 18;9(1):19368. doi: 10.1038/s41598-019-55900-6. Sci Rep. 2019. PMID: 31852969 Free PMC article.
-
Serotonin Receptor and Transporter Endocytosis Is an Important Factor in the Cellular Basis of Depression and Anxiety.Front Cell Neurosci. 2022 Feb 24;15:804592. doi: 10.3389/fncel.2021.804592. eCollection 2021. Front Cell Neurosci. 2022. PMID: 35280519 Free PMC article.
-
DYRK1-mediated phosphorylation of endocytic components is required for extracellular lumen expansion in ascidian notochord.Biol Res. 2023 Mar 11;56(1):10. doi: 10.1186/s40659-023-00422-9. Biol Res. 2023. PMID: 36899423 Free PMC article.
References
Publication types
MeSH terms
Substances
Grants and funding
LinkOut - more resources
Full Text Sources
Other Literature Sources
Research Materials
Miscellaneous