Methylation of lysine 9 in histone H3 directs alternative modes of highly dynamic interaction of heterochromatin protein hHP1β with the nucleosome
- PMID: 22815475
- PMCID: PMC3460472
- DOI: 10.1074/jbc.M112.390849
Methylation of lysine 9 in histone H3 directs alternative modes of highly dynamic interaction of heterochromatin protein hHP1β with the nucleosome
Abstract
Binding of heterochromatin protein 1 (HP1) to the histone H3 lysine 9 trimethylation (H3K9me3) mark is a hallmark of establishment and maintenance of heterochromatin. Although genetic and cell biological aspects have been elucidated, the molecular details of HP1 binding to H3K9me3 nucleosomes are unknown. Using a combination of NMR spectroscopy and biophysical measurements on fully defined recombinant experimental systems, we demonstrate that H3K9me3 works as an on/off switch regulating distinct binding modes of hHP1β to the nucleosome. The methyl-mark determines a highly flexible and very dynamic interaction of the chromodomain of hHP1β with the H3-tail. There are no other constraints of interaction or additional multimerization interfaces. In contrast, in the absence of methylation, the hinge region and the N-terminal tail form weak nucleosome contacts mainly with DNA. In agreement with the high flexibility within the hHP1β-H3K9me3 nucleosome complex, the chromoshadow domain does not provide a direct binding interface. Our results report the first detailed structural analysis of a dynamic protein-nucleosome complex directed by a histone modification and provide a conceptual framework for understanding similar interactions in the context of chromatin.
Figures
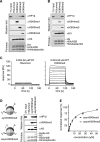
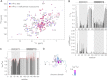
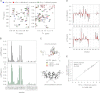
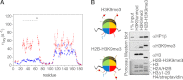
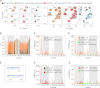
Similar articles
-
Dynamic and flexible H3K9me3 bridging via HP1β dimerization establishes a plastic state of condensed chromatin.Nat Commun. 2016 Apr 19;7:11310. doi: 10.1038/ncomms11310. Nat Commun. 2016. PMID: 27090491 Free PMC article.
-
Hinge and chromoshadow of HP1α participate in recognition of K9 methylated histone H3 in nucleosomes.J Mol Biol. 2013 Jan 9;425(1):54-70. doi: 10.1016/j.jmb.2012.10.018. Epub 2012 Nov 6. J Mol Biol. 2013. PMID: 23142645
-
A conformational switch in HP1 releases auto-inhibition to drive heterochromatin assembly.Nature. 2013 Apr 18;496(7445):377-81. doi: 10.1038/nature12032. Epub 2013 Mar 13. Nature. 2013. PMID: 23485968 Free PMC article.
-
Towards understanding methyllysine readout.Biochim Biophys Acta. 2014 Aug;1839(8):686-93. doi: 10.1016/j.bbagrm.2014.04.001. Epub 2014 Apr 13. Biochim Biophys Acta. 2014. PMID: 24727128 Free PMC article. Review.
-
Epigenetic regulation by histone methylation and histone variants.Mol Endocrinol. 2005 Mar;19(3):563-73. doi: 10.1210/me.2004-0496. Epub 2005 Jan 27. Mol Endocrinol. 2005. PMID: 15677708 Review.
Cited by
-
TGM2-mediated histone transglutamination is dictated by steric accessibility.Proc Natl Acad Sci U S A. 2022 Oct 25;119(43):e2208672119. doi: 10.1073/pnas.2208672119. Epub 2022 Oct 18. Proc Natl Acad Sci U S A. 2022. PMID: 36256821 Free PMC article.
-
HP1a: a structural chromosomal protein regulating transcription.Trends Genet. 2014 Mar;30(3):103-10. doi: 10.1016/j.tig.2014.01.002. Epub 2014 Feb 17. Trends Genet. 2014. PMID: 24555990 Free PMC article. Review.
-
Calibrating ChIP-Seq with Nucleosomal Internal Standards to Measure Histone Modification Density Genome Wide.Mol Cell. 2015 Jun 4;58(5):886-99. doi: 10.1016/j.molcel.2015.04.022. Epub 2015 May 21. Mol Cell. 2015. PMID: 26004229 Free PMC article.
-
Structural plasticity in human heterochromatin protein 1β.PLoS One. 2013 Apr 9;8(4):e60887. doi: 10.1371/journal.pone.0060887. Print 2013. PLoS One. 2013. PMID: 23585859 Free PMC article.
-
Histone H4 Tails in Nucleosomes: a Fuzzy Interaction with DNA.Angew Chem Int Ed Engl. 2021 Mar 15;60(12):6480-6487. doi: 10.1002/anie.202012046. Epub 2021 Feb 12. Angew Chem Int Ed Engl. 2021. PMID: 33522067 Free PMC article.
References
-
- Ebert A., Lein S., Schotta G., Reuter G. (2006) Histone modification and the control of heterochromatic gene silencing in Drosophila. Chromosome Res. 14, 377–392 - PubMed
-
- Ayyanathan K., Lechner M. S., Bell P., Maul G. G., Schultz D. C., Yamada Y., Tanaka K., Torigoe K., Rauscher F. J., 3rd (2003) Regulated recruitment of HP1 to a euchromatic gene induces mitotically heritable, epigenetic gene silencing. A mammalian cell culture model of gene variegation. Genes Dev. 17, 1855–1869 - PMC - PubMed
-
- Singh P. B. (2010) HP1 proteins. What is the essential interaction? Genetika 46, 1424–1429 - PubMed
Publication types
MeSH terms
Substances
LinkOut - more resources
Full Text Sources
Other Literature Sources
Molecular Biology Databases
Research Materials