DnaK chaperone-dependent disaggregation by caseinolytic peptidase B (ClpB) mutants reveals functional overlap in the N-terminal domain and nucleotide-binding domain-1 pore tyrosine
- PMID: 22745126
- PMCID: PMC3436547
- DOI: 10.1074/jbc.M112.383091
DnaK chaperone-dependent disaggregation by caseinolytic peptidase B (ClpB) mutants reveals functional overlap in the N-terminal domain and nucleotide-binding domain-1 pore tyrosine
Abstract
Protein disaggregation in Escherichia coli is carried out by ClpB, an AAA(+) (ATPases associated with various cellular activities) molecular chaperone, together with the DnaK chaperone system. Conformational changes in ClpB driven by ATP binding and hydrolysis promote substrate binding, unfolding, and translocation. Conserved pore tyrosines in both nucleotide-binding domain-1 (NBD-1) and -2 (NBD-2), which reside in flexible loops extending into the central pore of the ClpB hexamer, bind substrates. When the NBD-1 pore loop tyrosine is substituted with alanine (Y251A), ClpB can collaborate with the DnaK system in disaggregation, although activity is reduced. The N-domain has also been implicated in substrate binding, and like the NBD-1 pore loop tyrosine, it is not essential for disaggregation activity. To further probe the function and interplay of the ClpB N-domain and the NBD-1 pore loop, we made a double mutant with an N-domain deletion and a Y251A substitution. This ClpB double mutant is inactive in substrate disaggregation with the DnaK system, although each single mutant alone can function with DnaK. Our data suggest that this loss in activity is primarily due to a decrease in substrate engagement by ClpB prior to substrate unfolding and translocation and indicate an overlapping function for the N-domain and NBD-1 pore tyrosine. Furthermore, the functional overlap seen in the presence of the DnaK system is not observed in the absence of DnaK. For innate ClpB unfolding activity, the NBD-1 pore tyrosine is required, and the presence of the N-domain is insufficient to overcome the defect of the ClpB Y251A mutant.
Figures
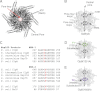
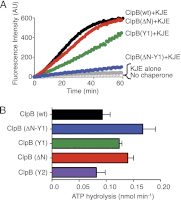
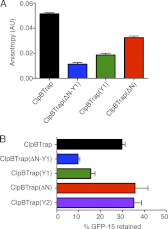
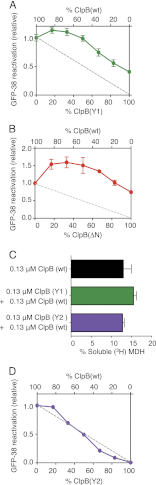
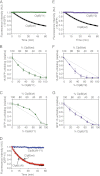
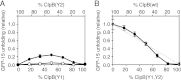
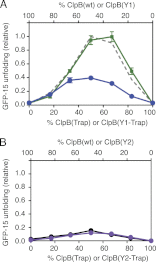
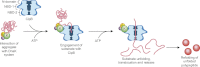
Similar articles
-
Interplay between E. coli DnaK, ClpB and GrpE during protein disaggregation.J Mol Biol. 2015 Jan 30;427(2):312-27. doi: 10.1016/j.jmb.2014.10.013. Epub 2014 Oct 29. J Mol Biol. 2015. PMID: 25451597 Free PMC article.
-
Species-specific collaboration of heat shock proteins (Hsp) 70 and 100 in thermotolerance and protein disaggregation.Proc Natl Acad Sci U S A. 2011 Apr 26;108(17):6915-20. doi: 10.1073/pnas.1102828108. Epub 2011 Apr 7. Proc Natl Acad Sci U S A. 2011. PMID: 21474779 Free PMC article.
-
Coupling ATP utilization to protein remodeling by ClpB, a hexameric AAA+ protein.Proc Natl Acad Sci U S A. 2009 Dec 29;106(52):22233-8. doi: 10.1073/pnas.0911937106. Epub 2009 Nov 25. Proc Natl Acad Sci U S A. 2009. PMID: 19940245 Free PMC article.
-
Reactivation of Aggregated Proteins by the ClpB/DnaK Bi-Chaperone System.Curr Protoc Protein Sci. 2016 Feb 2;83:28.10.1-28.10.18. doi: 10.1002/0471140864.ps2810s83. Curr Protoc Protein Sci. 2016. PMID: 26836408 Free PMC article. Review.
-
Novel insights into the mechanism of chaperone-assisted protein disaggregation.Biol Chem. 2005 Aug;386(8):739-44. doi: 10.1515/BC.2005.086. Biol Chem. 2005. PMID: 16201868 Review.
Cited by
-
The Cryo-EM Effect: Structural Biology of Neurodegenerative Disease Proteostasis Factors.J Neuropathol Exp Neurol. 2021 Jun 4;80(6):494-513. doi: 10.1093/jnen/nlab029. J Neuropathol Exp Neurol. 2021. PMID: 33860329 Free PMC article. Review.
-
Factors underlying asymmetric pore dynamics of disaggregase and microtubule-severing AAA+ machines.Biophys J. 2021 Aug 17;120(16):3437-3454. doi: 10.1016/j.bpj.2021.05.027. Epub 2021 Jun 25. Biophys J. 2021. PMID: 34181904 Free PMC article.
-
Substrate Discrimination by ClpB and Hsp104.Front Mol Biosci. 2017 May 29;4:36. doi: 10.3389/fmolb.2017.00036. eCollection 2017. Front Mol Biosci. 2017. PMID: 28611991 Free PMC article.
-
Heat shock protein (Hsp) 70 is an activator of the Hsp104 motor.Proc Natl Acad Sci U S A. 2013 May 21;110(21):8513-8. doi: 10.1073/pnas.1217988110. Epub 2013 May 6. Proc Natl Acad Sci U S A. 2013. PMID: 23650362 Free PMC article.
-
Microtubule-severing enzymes: From cellular functions to molecular mechanism.J Cell Biol. 2018 Dec 3;217(12):4057-4069. doi: 10.1083/jcb.201612104. Epub 2018 Oct 29. J Cell Biol. 2018. PMID: 30373906 Free PMC article. Review.
References
-
- Doyle S. M., Wickner S. (2009) Hsp104 and ClpB. Protein disaggregating machines. Trends Biochem. Sci. 34, 40–48 - PubMed
-
- Mogk A., Haslberger T., Tessarz P., Bukau B. (2008) Common and specific mechanisms of AAA+ proteins involved in protein quality control. Biochem. Soc. Trans. 36, 120–125 - PubMed
-
- Bukau B., Horwich A. L. (1998) The Hsp70 and Hsp60 chaperone machines. Cell 92, 351–366 - PubMed
Publication types
MeSH terms
Substances
Grants and funding
LinkOut - more resources
Full Text Sources
Molecular Biology Databases