FGFR1 cleavage and nuclear translocation regulates breast cancer cell behavior
- PMID: 22665522
- PMCID: PMC3373409
- DOI: 10.1083/jcb.201108077
FGFR1 cleavage and nuclear translocation regulates breast cancer cell behavior
Abstract
FGF-10 and its receptors, FGFR1 and FGFR2, have been implicated in breast cancer susceptibility and progression, suggesting that fibroblast growth factor (FGF) signaling may be co-opted by breast cancer cells. We identify a novel pathway downstream of FGFR1 activation, whereby the receptor is cleaved and traffics to the nucleus, where it can regulate specific target genes. We confirm Granzyme B (GrB) as the protease responsible for cleavage and show that blocking GrB activity stopped FGFR1 trafficking to the nucleus and abrogates the promigratory effect of FGF stimulation. We confirm the in vivo relevance of our findings, showing that FGFR1 localized to the nucleus specifically in invading cells in both clinical material and a three-dimensional model of breast cancer. We identify target genes for FGFR1, which exert significant effects on cell migration and may represent an invasive signature. Our experiments identify a novel mechanism by which FGF signaling can regulate cancer cell behavior and provide a novel therapeutic target for treatment of invasive breast cancer.
Figures
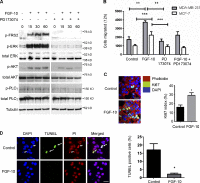
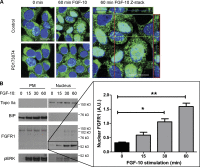
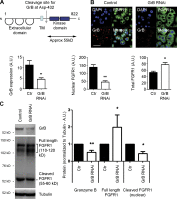
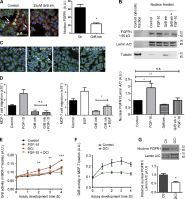
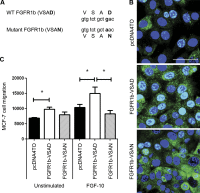
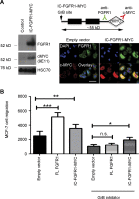
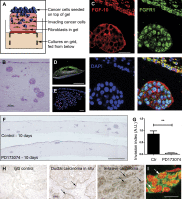
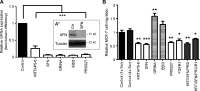
Similar articles
-
Differential roles of fibroblast growth factor receptors (FGFR) 1, 2 and 3 in the regulation of S115 breast cancer cell growth.PLoS One. 2012;7(11):e49970. doi: 10.1371/journal.pone.0049970. Epub 2012 Nov 21. PLoS One. 2012. PMID: 23185502 Free PMC article.
-
Fibroblast growth factor-2, derived from cancer-associated fibroblasts, stimulates growth and progression of human breast cancer cells via FGFR1 signaling.Mol Carcinog. 2020 Sep;59(9):1028-1040. doi: 10.1002/mc.23233. Epub 2020 Jun 18. Mol Carcinog. 2020. PMID: 32557854
-
TNFAIP3 is required for FGFR1 activation-promoted proliferation and tumorigenesis of premalignant DCIS.COM human mammary epithelial cells.Breast Cancer Res. 2018 Aug 15;20(1):97. doi: 10.1186/s13058-018-1024-9. Breast Cancer Res. 2018. PMID: 30111373 Free PMC article.
-
Integrative nuclear FGFR1 signaling (INFS) as a part of a universal "feed-forward-and-gate" signaling module that controls cell growth and differentiation.J Cell Biochem. 2003 Nov 1;90(4):662-91. doi: 10.1002/jcb.10606. J Cell Biochem. 2003. PMID: 14587025 Review.
-
Integrative nuclear signaling in cell development--a role for FGF receptor-1.DNA Cell Biol. 2007 Dec;26(12):811-26. doi: 10.1089/dna.2007.0664. DNA Cell Biol. 2007. PMID: 18021009 Review.
Cited by
-
Caffeic acid phenethyl ester suppresses metastasis of breast cancer cells by inactivating FGFR1 via MD2.PLoS One. 2023 Jul 25;18(7):e0289031. doi: 10.1371/journal.pone.0289031. eCollection 2023. PLoS One. 2023. PMID: 37490511 Free PMC article.
-
Engineering targeted chromosomal amplifications in human breast epithelial cells.Breast Cancer Res Treat. 2015 Jul;152(2):313-21. doi: 10.1007/s10549-015-3468-2. Epub 2015 Jun 23. Breast Cancer Res Treat. 2015. PMID: 26099605 Free PMC article.
-
Nuclear FGFR1 promotes pancreatic stellate cell-driven invasion through up-regulation of Neuregulin 1.Oncogene. 2023 Feb;42(7):491-500. doi: 10.1038/s41388-022-02513-5. Epub 2022 Nov 10. Oncogene. 2023. PMID: 36357571 Free PMC article.
-
The FGFR1 V561M Gatekeeper Mutation Drives AZD4547 Resistance through STAT3 Activation and EMT.Mol Cancer Res. 2019 Feb;17(2):532-543. doi: 10.1158/1541-7786.MCR-18-0429. Epub 2018 Sep 26. Mol Cancer Res. 2019. PMID: 30257990 Free PMC article.
-
A truncated derivative of FGFR1 kinase cooperates with FLT3 and KIT to transform hematopoietic stem cells in syndromic and de novo AML.Mol Cancer. 2022 Jul 29;21(1):156. doi: 10.1186/s12943-022-01628-3. Mol Cancer. 2022. PMID: 35906694 Free PMC article.
References
-
- Adam R.M., Danciu T., McLellan D.L., Borer J.G., Lin J., Zurakowski D., Weinstein M.H., Rajjayabun P.H., Mellon J.K., Freeman M.R. 2003. A nuclear form of the heparin-binding epidermal growth factor-like growth factor precursor is a feature of aggressive transitional cell carcinoma. Cancer Res. 63:484–490 - PubMed
-
- Badea L., Herlea V., Dima S.O., Dumitrascu T., Popescu I. 2008. Combined gene expression analysis of whole-tissue and microdissected pancreatic ductal adenocarcinoma identifies genes specifically overexpressed in tumor epithelia. Hepatogastroenterology. 55:2016–2027 - PubMed
Publication types
MeSH terms
Substances
Grants and funding
LinkOut - more resources
Full Text Sources
Medical
Miscellaneous