Quantitative analysis of prenylated RhoA interaction with its chaperone, RhoGDI
- PMID: 22628549
- PMCID: PMC3410996
- DOI: 10.1074/jbc.M112.371294
Quantitative analysis of prenylated RhoA interaction with its chaperone, RhoGDI
Abstract
Small GTPases of the Rho family regulate cytoskeleton remodeling, cell polarity, and transcription, as well as the cell cycle, in eukaryotic cells. Membrane delivery and recycling of the Rho GTPases is mediated by Rho GDP dissociation inhibitor (RhoGDI), which forms a stable complex with prenylated Rho GTPases. We analyzed the interaction of RhoGDI with the active and inactive forms of prenylated and unprenylated RhoA. We demonstrate that RhoGDI binds the prenylated form of RhoA·GDP with unexpectedly high affinity (K(d) = 5 pm). The very long half-life of the complex is reduced 25-fold on RhoA activation, with a concomitant reduction in affinity (K(d) = 3 nm). The 2.8-Å structure of the RhoA·guanosine 5'-[β,γ-imido] triphosphate (GMPPNP)·RhoGDI complex demonstrated that complex formation forces the activated RhoA into a GDP-bound conformation in the absence of nucleotide hydrolysis. We demonstrate that membrane extraction of Rho GTPase by RhoGDI is a thermodynamically favored passive process that operates through a series of progressively tighter intermediates, much like the one that is mediated by RabGDI.
Figures
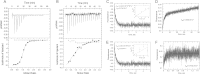
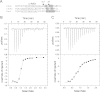
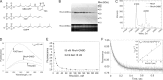
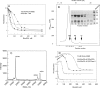
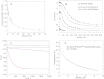
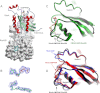
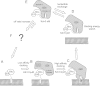
Similar articles
-
Effects of lovastatin on Rho isoform expression, activity, and association with guanine nucleotide dissociation inhibitors.Biochem Pharmacol. 2008 Jan 15;75(2):405-13. doi: 10.1016/j.bcp.2007.08.031. Epub 2007 Sep 1. Biochem Pharmacol. 2008. PMID: 17920041 Free PMC article.
-
Human RhoA/RhoGDI complex expressed in yeast: GTP exchange is sufficient for translocation of RhoA to liposomes.Protein Sci. 2000 Feb;9(2):376-86. doi: 10.1110/ps.9.2.376. Protein Sci. 2000. PMID: 10716190 Free PMC article.
-
Phosphorylation states of Cdc42 and RhoA regulate their interactions with Rho GDP dissociation inhibitor and their extraction from biological membranes.Biochem J. 2002 Jan 15;361(Pt 2):243-54. doi: 10.1042/0264-6021:3610243. Biochem J. 2002. PMID: 11772396 Free PMC article.
-
Rho guanine dissociation inhibitors: pivotal molecules in cellular signalling.Cell Signal. 1999 Aug;11(8):545-54. doi: 10.1016/s0898-6568(98)00063-1. Cell Signal. 1999. PMID: 10433515 Review.
-
RhoGDI: multiple functions in the regulation of Rho family GTPase activities.Biochem J. 2005 Aug 15;390(Pt 1):1-9. doi: 10.1042/BJ20050104. Biochem J. 2005. PMID: 16083425 Free PMC article. Review.
Cited by
-
A Complete Survey of RhoGDI Targets Reveals Novel Interactions with Atypical Small GTPases.Biochemistry. 2021 May 18;60(19):1533-1551. doi: 10.1021/acs.biochem.1c00120. Epub 2021 Apr 29. Biochemistry. 2021. PMID: 33913706 Free PMC article.
-
Crystal Structure of Schizosaccharomyces pombe Rho1 Reveals Its Evolutionary Relationship with Other Rho GTPases.Biology (Basel). 2022 Nov 7;11(11):1627. doi: 10.3390/biology11111627. Biology (Basel). 2022. PMID: 36358328 Free PMC article.
-
The Rho GTPase signalling pathway in urothelial carcinoma.Nat Rev Urol. 2018 Feb;15(2):83-91. doi: 10.1038/nrurol.2017.184. Epub 2017 Nov 14. Nat Rev Urol. 2018. PMID: 29133936 Review.
-
Reconstitution of a Reversible Membrane Switch via Prenylation by One-Pot Cell-Free Expression.ACS Synth Biol. 2023 Jan 20;12(1):108-119. doi: 10.1021/acssynbio.2c00406. Epub 2022 Nov 29. ACS Synth Biol. 2023. PMID: 36445320 Free PMC article.
-
Mechanistic insights into the role of prenyl-binding protein PrBP/δ in membrane dissociation of phosphodiesterase 6.Nat Commun. 2018 Jan 8;9(1):90. doi: 10.1038/s41467-017-02569-y. Nat Commun. 2018. PMID: 29311697 Free PMC article.
References
-
- Ridley A. J., Paterson H. F., Johnston C. L., Diekmann D., Hall A. (1992) The small GTP-binding protein Rac regulates growth factor-induced membrane ruffling. Cell 70, 401–410 - PubMed
-
- Yamana N., Arakawa Y., Nishino T., Kurokawa K., Tanji M., Itoh R. E., Monypenny J., Ishizaki T., Bito H., Nozaki K., Hashimoto N., Matsuda M., Narumiya S. (2006) The Rho-mDia1 pathway regulates cell polarity and focal adhesion turnover in migrating cells through mobilizing Apc and c-Src. Mol. Cell. Biol. 26, 6844–6858 - PMC - PubMed
-
- Hill C. S., Wynne J., Treisman R. (1995) The Rho family GTPases RhoA, Rac1, and CDC42Hs regulate transcriptional activation by SRF. Cell 81, 1159–1170 - PubMed
-
- Schmidt A., Hall A. (2002) Guanine nucleotide exchange factors for Rho GTPases. Turning on the switch. Genes Dev. 16, 1587–1609 - PubMed
Publication types
MeSH terms
Substances
Associated data
- Actions
LinkOut - more resources
Full Text Sources
Molecular Biology Databases