β-Catenin gain of function in muscles impairs neuromuscular junction formation
- PMID: 22627288
- PMCID: PMC3367446
- DOI: 10.1242/dev.080705
β-Catenin gain of function in muscles impairs neuromuscular junction formation
Erratum in
- Development. 2012 Jul;139(14):2636
Abstract
Neuromuscular junction (NMJ) formation requires proper interaction between motoneurons and muscle cells. β-Catenin is required in muscle cells for NMJ formation. To understand underlying mechanisms, we investigated the effect of β-catenin gain of function (GOF) on NMJ development. In HSA-β-cat(flox(ex3)/+) mice, which express stable β-catenin specifically in muscles, motor nerve terminals became extensively defasciculated and arborized. Ectopic muscles were observed in the diaphragm and were innervated by ectopic phrenic nerve branches. Moreover, extensive outgrowth and branching of spinal axons were evident in the GOF mice. These results indicate that increased β-catenin in muscles alters presynaptic differentiation. Postsynaptically, AChR clusters in HSA-β-cat(flox(ex3)/+) diaphragms were distributed in a wider region, suggesting that muscle β-catenin GOF disrupted the signal that restricts AChR clustering to the middle region of muscle fibers. Expression of stable β-catenin in motoneurons, however, had no effect on NMJ formation. These observations provide additional genetic evidence that pre- and postsynaptic development of the NMJ requires an intricate balance of β-catenin activity in muscles.
Figures
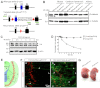
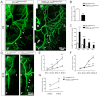
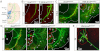
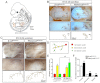
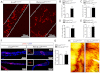
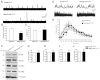
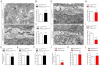
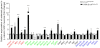
Similar articles
-
Muscle Yap Is a Regulator of Neuromuscular Junction Formation and Regeneration.J Neurosci. 2017 Mar 29;37(13):3465-3477. doi: 10.1523/JNEUROSCI.2934-16.2017. Epub 2017 Feb 17. J Neurosci. 2017. PMID: 28213440 Free PMC article.
-
Transcriptome profile of subsynaptic myonuclei at the neuromuscular junction in embryogenesis.J Neurochem. 2024 Apr;168(4):342-354. doi: 10.1111/jnc.16013. Epub 2023 Nov 23. J Neurochem. 2024. PMID: 37994470
-
Retrograde regulation of motoneuron differentiation by muscle beta-catenin.Nat Neurosci. 2008 Mar;11(3):262-8. doi: 10.1038/nn2053. Epub 2008 Feb 17. Nat Neurosci. 2008. PMID: 18278041
-
Signaling and aging at the neuromuscular synapse: lessons learnt from neuromuscular diseases.Curr Opin Pharmacol. 2012 Jun;12(3):340-6. doi: 10.1016/j.coph.2012.02.002. Epub 2012 Feb 23. Curr Opin Pharmacol. 2012. PMID: 22365504 Review.
-
Development of the neuromuscular junction.Cell Tissue Res. 2006 Nov;326(2):263-71. doi: 10.1007/s00441-006-0237-x. Epub 2006 Jul 4. Cell Tissue Res. 2006. PMID: 16819627 Review.
Cited by
-
MuSK frizzled-like domain is critical for mammalian neuromuscular junction formation and maintenance.J Neurosci. 2015 Mar 25;35(12):4926-41. doi: 10.1523/JNEUROSCI.3381-14.2015. J Neurosci. 2015. PMID: 25810523 Free PMC article.
-
Differential effects of spinal motor neuron-derived and skeletal muscle-derived Rspo2 on acetylcholine receptor clustering at the neuromuscular junction.Sci Rep. 2018 Sep 11;8(1):13577. doi: 10.1038/s41598-018-31949-7. Sci Rep. 2018. PMID: 30206360 Free PMC article.
-
A novel ALS-associated variant in UBQLN4 regulates motor axon morphogenesis.Elife. 2017 May 2;6:e25453. doi: 10.7554/eLife.25453. Elife. 2017. PMID: 28463112 Free PMC article.
-
Transferrin receptor 1 plays an important role in muscle development and denervation-induced muscular atrophy.Neural Regen Res. 2021 Jul;16(7):1308-1316. doi: 10.4103/1673-5374.301024. Neural Regen Res. 2021. PMID: 33318410 Free PMC article.
-
The YAP1/TAZ-TEAD transcriptional network regulates gene expression at neuromuscular junctions in skeletal muscle fibers.Nucleic Acids Res. 2024 Jan 25;52(2):600-624. doi: 10.1093/nar/gkad1124. Nucleic Acids Res. 2024. PMID: 38048326 Free PMC article.
References
-
- Arber S., Han B., Mendelsohn M., Smith M., Jessell T. M., Sockanathan S. (1999). Requirement for the homeobox gene Hb9 in the consolidation of motor neuron identity. Neuron 23, 659–674 - PubMed
-
- Arikawa-Hirasawa E., Rossi S. G., Rotundo R. L., Yamada Y. (2002). Absence of acetylcholinesterase at the neuromuscular junctions of perlecan-null mice. Nat. Neurosci. 5, 119–123 - PubMed
-
- Bagri A., Cheng H. J., Yaron A., Pleasure S. J., Tessier-Lavigne M. (2003). Stereotyped pruning of long hippocampal axon branches triggered by retraction inducers of the semaphorin family. Cell 113, 285–299 - PubMed
Publication types
MeSH terms
Substances
Grants and funding
LinkOut - more resources
Full Text Sources
Other Literature Sources
Molecular Biology Databases
Research Materials
Miscellaneous