Induction of GADD34 is necessary for dsRNA-dependent interferon-β production and participates in the control of Chikungunya virus infection
- PMID: 22615568
- PMCID: PMC3355096
- DOI: 10.1371/journal.ppat.1002708
Induction of GADD34 is necessary for dsRNA-dependent interferon-β production and participates in the control of Chikungunya virus infection
Abstract
Nucleic acid sensing by cells is a key feature of antiviral responses, which generally result in type-I Interferon production and tissue protection. However, detection of double-stranded RNAs in virus-infected cells promotes two concomitant and apparently conflicting events. The dsRNA-dependent protein kinase (PKR) phosphorylates translation initiation factor 2-alpha (eIF2α) and inhibits protein synthesis, whereas cytosolic DExD/H box RNA helicases induce expression of type I-IFN and other cytokines. We demonstrate that the phosphatase-1 cofactor, growth arrest and DNA damage-inducible protein 34 (GADD34/Ppp1r15a), an important component of the unfolded protein response (UPR), is absolutely required for type I-IFN and IL-6 production by mouse embryonic fibroblasts (MEFs) in response to dsRNA. GADD34 expression in MEFs is dependent on PKR activation, linking cytosolic microbial sensing with the ATF4 branch of the UPR. The importance of this link for anti-viral immunity is underlined by the extreme susceptibility of GADD34-deficient fibroblasts and neonate mice to Chikungunya virus infection.
Conflict of interest statement
The authors have declared that no competing interests exist.
Figures
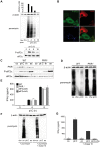
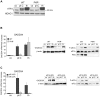
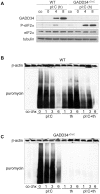
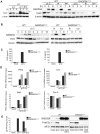
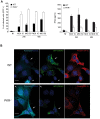
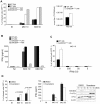
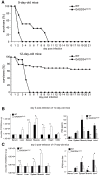
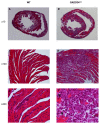
Similar articles
-
Protein synthesis inhibition and GADD34 control IFN-β heterogeneous expression in response to dsRNA.EMBO J. 2017 Mar 15;36(6):761-782. doi: 10.15252/embj.201695000. Epub 2017 Jan 18. EMBO J. 2017. PMID: 28100675 Free PMC article.
-
Regulation of de novo translation of host cells by manipulation of PERK/PKR and GADD34-PP1 activity during Newcastle disease virus infection.J Gen Virol. 2016 Apr;97(4):867-879. doi: 10.1099/jgv.0.000426. Epub 2016 Feb 11. J Gen Virol. 2016. PMID: 26869028
-
Protein phosphatase 1 subunit Ppp1r15a/GADD34 regulates cytokine production in polyinosinic:polycytidylic acid-stimulated dendritic cells.Proc Natl Acad Sci U S A. 2012 Feb 21;109(8):3006-11. doi: 10.1073/pnas.1104491109. Epub 2012 Feb 6. Proc Natl Acad Sci U S A. 2012. PMID: 22315398 Free PMC article.
-
Interferon β (IFN-β) Production during the Double-stranded RNA (dsRNA) Response in Hepatocytes Involves Coordinated and Feedforward Signaling through Toll-like Receptor 3 (TLR3), RNA-dependent Protein Kinase (PKR), Inducible Nitric Oxide Synthase (iNOS), and Src Protein.J Biol Chem. 2016 Jul 15;291(29):15093-107. doi: 10.1074/jbc.M116.717942. Epub 2016 May 17. J Biol Chem. 2016. PMID: 27226571 Free PMC article.
-
The PPP1R15 Family of eIF2-alpha Phosphatase Targeting Subunits (GADD34 and CReP).Int J Mol Sci. 2023 Dec 10;24(24):17321. doi: 10.3390/ijms242417321. Int J Mol Sci. 2023. PMID: 38139150 Free PMC article. Review.
Cited by
-
Induction of the Proinflammatory Chemokine Interleukin-8 Is Regulated by Integrated Stress Response and AP-1 Family Proteins Activated during Coronavirus Infection.Int J Mol Sci. 2021 May 26;22(11):5646. doi: 10.3390/ijms22115646. Int J Mol Sci. 2021. PMID: 34073283 Free PMC article.
-
Distinct Cellular Tropism and Immune Responses to Alphavirus Infection.Annu Rev Immunol. 2022 Apr 26;40:615-649. doi: 10.1146/annurev-immunol-101220-014952. Epub 2022 Feb 8. Annu Rev Immunol. 2022. PMID: 35134315 Free PMC article. Review.
-
Honokiol inhibits breast cancer cell metastasis by blocking EMT through modulation of Snail/Slug protein translation.Acta Pharmacol Sin. 2019 Sep;40(9):1219-1227. doi: 10.1038/s41401-019-0240-x. Epub 2019 Jun 24. Acta Pharmacol Sin. 2019. PMID: 31235819 Free PMC article.
-
Lower temperatures reduce type I interferon activity and promote alphaviral arthritis.PLoS Pathog. 2017 Dec 27;13(12):e1006788. doi: 10.1371/journal.ppat.1006788. eCollection 2017 Dec. PLoS Pathog. 2017. PMID: 29281739 Free PMC article.
-
Regulation of Cytokine Production by the Unfolded Protein Response; Implications for Infection and Autoimmunity.Front Immunol. 2018 Mar 5;9:422. doi: 10.3389/fimmu.2018.00422. eCollection 2018. Front Immunol. 2018. PMID: 29556237 Free PMC article. Review.
References
-
- Kawai T, Akira S. Innate immune recognition of viral infection. Nat Immunol. 2006;7:131–137. - PubMed
-
- Akira S, Uematsu S, Takeuchi O. Pathogen recognition and innate immunity. Cell. 2006;124:783–801. - PubMed
-
- Garcia MA, Meurs EF, Esteban M. The dsRNA protein kinase PKR: virus and cell control. Biochimie. 2007;89:799–811. - PubMed
Publication types
MeSH terms
Substances
LinkOut - more resources
Full Text Sources
Other Literature Sources
Medical
Molecular Biology Databases