Global analysis of chaperone effects using a reconstituted cell-free translation system
- PMID: 22615364
- PMCID: PMC3384135
- DOI: 10.1073/pnas.1201380109
Global analysis of chaperone effects using a reconstituted cell-free translation system
Abstract
Protein folding is often hampered by protein aggregation, which can be prevented by a variety of chaperones in the cell. A dataset that evaluates which chaperones are effective for aggregation-prone proteins would provide an invaluable resource not only for understanding the roles of chaperones, but also for broader applications in protein science and engineering. Therefore, we comprehensively evaluated the effects of the major Escherichia coli chaperones, trigger factor, DnaK/DnaJ/GrpE, and GroEL/GroES, on ∼800 aggregation-prone cytosolic E. coli proteins, using a reconstituted chaperone-free translation system. Statistical analyses revealed the robustness and the intriguing properties of chaperones. The DnaK and GroEL systems drastically increased the solubilities of hundreds of proteins with weak biases, whereas trigger factor had only a marginal effect on solubility. The combined addition of the chaperones was effective for a subset of proteins that were not rescued by any single chaperone system, supporting the synergistic effect of these chaperones. The resource, which is accessible via a public database, can be used to investigate the properties of proteins of interest in terms of their solubilities and chaperone effects.
Conflict of interest statement
The authors declare no conflict of interest.
Figures
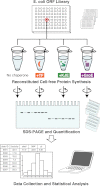
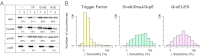
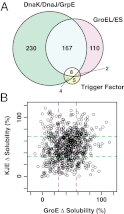
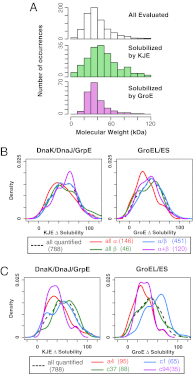
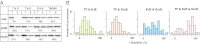
Similar articles
-
Interferon-gamma is a target for binding and folding by both Escherichia coli chaperone model systems GroEL/GroES and DnaK/DnaJ/GrpE.Biochimie. 1998 Aug-Sep;80(8-9):729-37. doi: 10.1016/s0300-9084(99)80026-1. Biochimie. 1998. PMID: 9865495 Review.
-
Chaperone-assisted protein folding in the cell cytoplasm.Curr Protein Pept Sci. 2001 Sep;2(3):227-44. doi: 10.2174/1389203013381134. Curr Protein Pept Sci. 2001. PMID: 12369934 Review.
-
ClpB and HtpG facilitate de novo protein folding in stressed Escherichia coli cells.Mol Microbiol. 2000 Jun;36(6):1360-70. doi: 10.1046/j.1365-2958.2000.01951.x. Mol Microbiol. 2000. PMID: 10931286
-
In vivo analysis of the overlapping functions of DnaK and trigger factor.EMBO Rep. 2004 Feb;5(2):195-200. doi: 10.1038/sj.embor.7400067. Epub 2004 Jan 9. EMBO Rep. 2004. PMID: 14726952 Free PMC article.
-
Recognizability of heterologous co-chaperones with Streptococcus intermedius DnaK and Escherichia coli DnaK.Microbiol Immunol. 2018 Nov;62(11):681-693. doi: 10.1111/1348-0421.12651. Epub 2018 Nov 5. Microbiol Immunol. 2018. PMID: 30239035
Cited by
-
Comparative Proteomic Analysis of Psychrophilic vs. Mesophilic Bacterial Species Reveals Different Strategies to Achieve Temperature Adaptation.Front Microbiol. 2022 May 3;13:841359. doi: 10.3389/fmicb.2022.841359. eCollection 2022. Front Microbiol. 2022. PMID: 35591995 Free PMC article.
-
Autonomous aggregation suppression by acidic residues explains why chaperones favour basic residues.EMBO J. 2020 Jun 2;39(11):e102864. doi: 10.15252/embj.2019102864. Epub 2020 Apr 1. EMBO J. 2020. PMID: 32237079 Free PMC article.
-
Heterologous expression of naturally evolved putative de novo proteins with chaperones.Protein Sci. 2022 Aug;31(8):e4371. doi: 10.1002/pro.4371. Protein Sci. 2022. PMID: 35900020 Free PMC article.
-
Critical Beginnings: Selective Tuning of Solubility and Structural Accuracy of Newly Synthesized Proteins by the Hsp70 Chaperone System.J Phys Chem B. 2023 May 11;127(18):3990-4014. doi: 10.1021/acs.jpcb.2c08485. Epub 2023 May 2. J Phys Chem B. 2023. PMID: 37130318 Free PMC article.
-
Cooperative working of bacterial chromosome replication proteins generated by a reconstituted protein expression system.Nucleic Acids Res. 2013 Aug;41(14):7176-83. doi: 10.1093/nar/gkt489. Epub 2013 Jun 3. Nucleic Acids Res. 2013. PMID: 23737447 Free PMC article.
References
-
- Dobson CM. Protein folding and misfolding. Nature. 2003;426:884–890. - PubMed
-
- Anfinsen CB. Principles that govern the folding of protein chains. Science. 1973;181:223–230. - PubMed
-
- Tyedmers J, Mogk A, Bukau B. Cellular strategies for controlling protein aggregation. Nat Rev Mol Cell Biol. 2010;11:777–788. - PubMed
-
- Hartl FU, Bracher A, Hayer-Hartl M. Molecular chaperones in protein folding and proteostasis. Nature. 2011;475:324–332. - PubMed
-
- Ellis J. Proteins as molecular chaperones. Nature. 1987;328:378–379. - PubMed
Publication types
MeSH terms
Substances
LinkOut - more resources
Full Text Sources
Other Literature Sources
Molecular Biology Databases
Research Materials