Pericytes support neutrophil subendothelial cell crawling and breaching of venular walls in vivo
- PMID: 22615129
- PMCID: PMC3371725
- DOI: 10.1084/jem.20111622
Pericytes support neutrophil subendothelial cell crawling and breaching of venular walls in vivo
Abstract
Neutrophil transmigration through venular walls that are composed of endothelial cells (ECs), pericytes, and the venular basement membrane is a key component of innate immunity. Through direct analysis of leukocyte-pericyte interactions in inflamed tissues using confocal intravital microscopy, we show how pericytes facilitate transmigration in vivo. After EC migration, neutrophils crawl along pericyte processes to gaps between adjacent pericytes in an ICAM-1-, Mac-1-, and LFA-1-dependent manner. These gaps were enlarged in inflamed tissues through pericyte shape change and were used as exit points by neutrophils in breaching the venular wall. The findings identify previously unknown roles for pericytes in neutrophil transmigration in vivo and add additional steps to the leukocyte adhesion cascade that supports leukocyte trafficking into sites of inflammation.
Figures
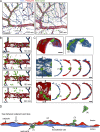
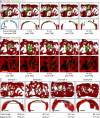
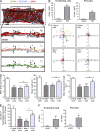
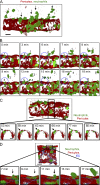
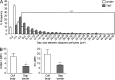
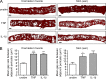
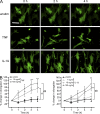
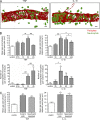

Similar articles
-
Pericytes regulate vascular basement membrane remodeling and govern neutrophil extravasation during inflammation.PLoS One. 2012;7(9):e45499. doi: 10.1371/journal.pone.0045499. Epub 2012 Sep 21. PLoS One. 2012. PMID: 23029055 Free PMC article.
-
Transendothelial migration enables subsequent transmigration of neutrophils through underlying pericytes.PLoS One. 2013;8(3):e60025. doi: 10.1371/journal.pone.0060025. Epub 2013 Mar 26. PLoS One. 2013. PMID: 23555870 Free PMC article.
-
Ultra structure analysis of cell-cell interactions between pericytes and neutrophils in vitro.Biochem Biophys Res Commun. 2014 Feb 28;445(1):180-3. doi: 10.1016/j.bbrc.2014.01.159. Epub 2014 Feb 1. Biochem Biophys Res Commun. 2014. PMID: 24495804
-
Role and Molecular Mechanisms of Pericytes in Regulation of Leukocyte Diapedesis in Inflamed Tissues.Mediators Inflamm. 2019 May 7;2019:4123605. doi: 10.1155/2019/4123605. eCollection 2019. Mediators Inflamm. 2019. PMID: 31205449 Free PMC article. Review.
-
Neutrophil transmigration: emergence of an adhesive cascade within venular walls.J Innate Immun. 2013;5(4):336-47. doi: 10.1159/000346659. Epub 2013 Mar 2. J Innate Immun. 2013. PMID: 23466407 Free PMC article. Review.
Cited by
-
Triple co-culture and perfusion bioreactor for studying the interaction between Neisseria gonorrhoeae and neutrophils: A novel 3D tissue model for bacterial infection and immunity.J Tissue Eng. 2021 Jan 28;12:2041731420988802. doi: 10.1177/2041731420988802. eCollection 2021 Jan-Dec. J Tissue Eng. 2021. PMID: 33796248 Free PMC article.
-
Characterization of Proteome Changes in Aged and Collagen VI-Deficient Human Pericyte Cultures.Int J Mol Sci. 2024 Jun 28;25(13):7118. doi: 10.3390/ijms25137118. Int J Mol Sci. 2024. PMID: 39000224 Free PMC article.
-
Learning in motion: pericytes instruct migrating innate leukocytes.Nat Immunol. 2013 Jan;14(1):14-5. doi: 10.1038/ni.2489. Nat Immunol. 2013. PMID: 23238752 No abstract available.
-
Ablation of Pericyte-Like Cells in Lungs by Oropharyngeal Aspiration of Diphtheria Toxin.Am J Respir Cell Mol Biol. 2017 Feb;56(2):160-167. doi: 10.1165/rcmb.2016-0083MA. Am J Respir Cell Mol Biol. 2017. PMID: 27779900 Free PMC article.
-
Laminin isoforms in endothelial and perivascular basement membranes.Cell Adh Migr. 2013 Jan-Feb;7(1):101-10. doi: 10.4161/cam.22680. Epub 2012 Dec 21. Cell Adh Migr. 2013. PMID: 23263631 Free PMC article. Review.
References
-
- Burns A.R., Walker D.C., Brown E.S., Thurmon L.T., Bowden R.A., Keese C.R., Simon S.I., Entman M.L., Smith C.W. 1997. Neutrophil transendothelial migration is independent of tight junctions and occurs preferentially at tricellular corners. J. Immunol. 159:2893–2903 - PubMed
-
- Christofidou-Solomidou M., Nakada M.T., Williams J., Muller W.A., DeLisser H.M. 1997. Neutrophil platelet endothelial cell adhesion molecule-1 participates in neutrophil recruitment at inflammatory sites and is down-regulated after leukocyte extravasation. J. Immunol. 158:4872–4878 - PubMed
-
- Cohen M.P., Frank R.N., Khalifa A.A. 1980. Collagen production by cultured retinal capillary pericytes. Invest. Ophthalmol. Vis. Sci. 19:90–94 - PubMed
Publication types
MeSH terms
Substances
Grants and funding
LinkOut - more resources
Full Text Sources
Other Literature Sources
Molecular Biology Databases
Research Materials
Miscellaneous