The origin and function of platelet glycosyltransferases
- PMID: 22613794
- PMCID: PMC3401214
- DOI: 10.1182/blood-2012-02-409235
The origin and function of platelet glycosyltransferases
Abstract
Platelets are megakaryocyte subfragments that participate in hemostatic and host defense reactions and deliver pro- and antiangiogenic factors throughout the vascular system. Although they are anucleated cells that lack a complex secretory apparatus with distinct Golgi/endoplasmic reticulum compartments, past studies have shown that platelets have glycosyltransferase activities. In the present study, we show that members of 3 distinct glycosyltransferase families are found within and on the surface of platelets. Immunocytology and flow cytometry results indicated that megakaryocytes package these Golgi-derived glycosyltransferases into vesicles that are sent via proplatelets to nascent platelets, where they accumulate. These glycosyltransferases are active, and intact platelets glycosylate large exogenous substrates. Furthermore, we show that activation of platelets results in the release of soluble glycosyltransferase activities and that platelets contain sufficient levels of sugar nucleotides for detection of glycosylation of exogenously added substrates. Therefore, the results of the present study show that blood platelets are a rich source of both glycosyltransferases and donor sugar substrates that can be released to function in the extracellular space. This platelet-glycosylation machinery offers a pathway to a simple glycoengineering strategy improving storage of platelets and may serve hitherto unknown biologic functions.
Figures
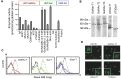
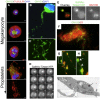
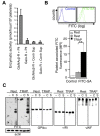
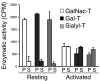
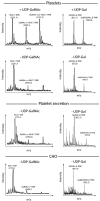
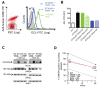
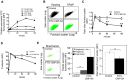
Similar articles
-
Platelets support extracellular sialylation by supplying the sugar donor substrate.J Biol Chem. 2014 Mar 28;289(13):8742-8. doi: 10.1074/jbc.C113.546713. Epub 2014 Feb 18. J Biol Chem. 2014. PMID: 24550397 Free PMC article.
-
Circulating blood and platelets supply glycosyltransferases that enable extrinsic extracellular glycosylation.Glycobiology. 2017 Jan;27(2):188-198. doi: 10.1093/glycob/cww108. Epub 2016 Oct 26. Glycobiology. 2017. PMID: 27798070 Free PMC article.
-
Ganglioside glycosyltransferases organize in distinct multienzyme complexes in CHO-K1 cells.J Biol Chem. 2003 Oct 10;278(41):40262-71. doi: 10.1074/jbc.M305455200. Epub 2003 Aug 4. J Biol Chem. 2003. PMID: 12900410
-
The serendipity of ganglioside biosynthesis: pathway to CARS and HY-CARS glycosyltransferases.Glycobiology. 1991 Nov;1(5):469-75. doi: 10.1093/glycob/1.5.469. Glycobiology. 1991. PMID: 1822228 Review. No abstract available.
-
Golgi localization of glycosyltransferases: more questions than answers.Glycobiology. 1997 Feb;7(1):1-13. doi: 10.1093/glycob/7.1.1-b. Glycobiology. 1997. PMID: 9061359 Free PMC article. Review.
Cited by
-
Sialic acid in the regulation of blood cell production, differentiation and turnover.Immunology. 2024 Aug;172(4):517-532. doi: 10.1111/imm.13780. Epub 2024 Mar 19. Immunology. 2024. PMID: 38503445 Review.
-
Mucin-Type O-GalNAc Glycosylation in Health and Disease.Adv Exp Med Biol. 2021;1325:25-60. doi: 10.1007/978-3-030-70115-4_2. Adv Exp Med Biol. 2021. PMID: 34495529
-
Inherited Thrombocytopenia Caused by Variants in Crucial Genes for Glycosylation.Int J Mol Sci. 2023 Mar 7;24(6):5109. doi: 10.3390/ijms24065109. Int J Mol Sci. 2023. PMID: 36982178 Free PMC article. Review.
-
The intracellular and plasma membrane pools of phosphatidylinositol-4-monophosphate control megakaryocyte maturation and proplatelet formation.Res Pract Thromb Haemost. 2023 Apr 26;7(4):100169. doi: 10.1016/j.rpth.2023.100169. eCollection 2023 May. Res Pract Thromb Haemost. 2023. PMID: 37304829 Free PMC article.
-
Trp53 regulates platelets in bone marrow via the PI3K pathway.Exp Ther Med. 2020 Aug;20(2):1253-1260. doi: 10.3892/etm.2020.8850. Epub 2020 Jun 9. Exp Ther Med. 2020. PMID: 32765666 Free PMC article.
References
-
- Colley KJ, Lee EU, Paulson JC. The signal anchor and stem regions of the beta-galactoside alpha 2,6-sialyltransferase may each act to localize the enzyme to the Golgi apparatus. J Biol Chem. 1992;267(11):7784–7793. - PubMed
-
- Nilsson T, Au CE, Bergeron JJ. Sorting out glycosylation enzymes in the Golgi apparatus. FEBS Lett. 2009;583(23):3764–3769. - PubMed
-
- Paulson JC, Colley KJ. Glycosyltransferases. Structure, localization, and control of cell type-specific glycosylation. J Biol Chem. 1989;264(30):17615–17618. - PubMed
-
- Berger EG. Ectopic localizations of Golgi glycosyltransferases. Glycobiology. 2002;12(2):29R–36R. - PubMed
Publication types
MeSH terms
Substances
Grants and funding
LinkOut - more resources
Full Text Sources
Other Literature Sources
Molecular Biology Databases
Miscellaneous