Cavities determine the pressure unfolding of proteins
- PMID: 22496593
- PMCID: PMC3344970
- DOI: 10.1073/pnas.1200915109
Cavities determine the pressure unfolding of proteins
Abstract
It has been known for nearly 100 years that pressure unfolds proteins, yet the physical basis of this effect is not understood. Unfolding by pressure implies that the molar volume of the unfolded state of a protein is smaller than that of the folded state. This decrease in volume has been proposed to arise from differences between the density of bulk water and water associated with the protein, from pressure-dependent changes in the structure of bulk water, from the loss of internal cavities in the folded states of proteins, or from some combination of these three factors. Here, using 10 cavity-containing variants of staphylococcal nuclease, we demonstrate that pressure unfolds proteins primarily as a result of cavities that are present in the folded state and absent in the unfolded one. High-pressure NMR spectroscopy and simulations constrained by the NMR data were used to describe structural and energetic details of the folding landscape of staphylococcal nuclease that are usually inaccessible with existing experimental approaches using harsher denaturants. Besides solving a 100-year-old conundrum concerning the detailed structural origins of pressure unfolding of proteins, these studies illustrate the promise of pressure perturbation as a unique tool for examining the roles of packing, conformational fluctuations, and water penetration as determinants of solution properties of proteins, and for detecting folding intermediates and other structural details of protein-folding landscapes that are invisible to standard experimental approaches.
Conflict of interest statement
The authors declare no conflict of interest.
Figures
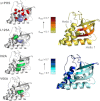
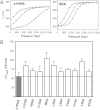
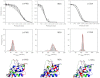
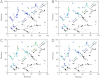
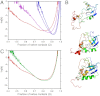
Comment in
-
Proteins under pressure.Proc Natl Acad Sci U S A. 2012 May 1;109(18):6792-3. doi: 10.1073/pnas.1204795109. Epub 2012 Apr 23. Proc Natl Acad Sci U S A. 2012. PMID: 22529364 Free PMC article. No abstract available.
Similar articles
-
High-pressure denaturation of staphylococcal nuclease proline-to-glycine substitution mutants.Biochemistry. 1996 Mar 26;35(12):3857-64. doi: 10.1021/bi952012g. Biochemistry. 1996. PMID: 8620010
-
A hypothesis to reconcile the physical and chemical unfolding of proteins.Proc Natl Acad Sci U S A. 2015 May 26;112(21):E2775-84. doi: 10.1073/pnas.1500352112. Epub 2015 May 11. Proc Natl Acad Sci U S A. 2015. PMID: 25964355 Free PMC article.
-
Studying pressure denaturation of a protein by molecular dynamics simulations.Proteins. 2010 May 15;78(7):1641-51. doi: 10.1002/prot.22680. Proteins. 2010. PMID: 20146357
-
Intermediate states in protein folding.J Mol Biol. 1996 May 24;258(5):707-25. doi: 10.1006/jmbi.1996.0280. J Mol Biol. 1996. PMID: 8637003 Review.
-
Engineering more stable proteins.Chem Soc Rev. 2018 Dec 21;47(24):9026-9045. doi: 10.1039/c8cs00014j. Epub 2018 Oct 11. Chem Soc Rev. 2018. PMID: 30306986 Review.
Cited by
-
Misplaced helix slows down ultrafast pressure-jump protein folding.Proc Natl Acad Sci U S A. 2013 May 14;110(20):8087-92. doi: 10.1073/pnas.1219163110. Epub 2013 Apr 25. Proc Natl Acad Sci U S A. 2013. PMID: 23620522 Free PMC article.
-
Pressure and Chemical Unfolding of an α-Helical Bundle Protein: The GH2 Domain of the Protein Adaptor GIPC1.Int J Mol Sci. 2021 Mar 30;22(7):3597. doi: 10.3390/ijms22073597. Int J Mol Sci. 2021. PMID: 33808390 Free PMC article.
-
Probing the structural stability of R-phycocyanin under pressure.Protein Sci. 2024 Sep;33(9):e5145. doi: 10.1002/pro.5145. Protein Sci. 2024. PMID: 39150070
-
Heat and cold denaturation of yeast frataxin: The effect of pressure.Biophys J. 2022 Apr 19;121(8):1502-1511. doi: 10.1016/j.bpj.2022.03.010. Epub 2022 Mar 9. Biophys J. 2022. PMID: 35278425 Free PMC article.
-
Dissecting the contributions of β-hairpin tyrosine pairs to the folding and stability of long-lived human γD-crystallins.Nanoscale. 2014;6(3):1797-807. doi: 10.1039/c3nr03782g. Nanoscale. 2014. PMID: 24352614 Free PMC article.
References
-
- Bridgman PW. The coagulation of albumin by pressure. J Biol Chem. 1914;19:511–512.
-
- Chalikian TV, Macgregor RB., Jr Origins of pressure-induced protein transitions. J Mol Biol. 2009;394:834–842. - PubMed
Publication types
MeSH terms
Substances
Associated data
- Actions
- Actions
- Actions
- Actions
- Actions
- Actions
- Actions
- Actions
- Actions
- Actions
- Actions
- Actions
Grants and funding
LinkOut - more resources
Full Text Sources