The eukaryotic initiation factor eIF4H facilitates loop-binding, repetitive RNA unwinding by the eIF4A DEAD-box helicase
- PMID: 22457067
- PMCID: PMC3401463
- DOI: 10.1093/nar/gks278
The eukaryotic initiation factor eIF4H facilitates loop-binding, repetitive RNA unwinding by the eIF4A DEAD-box helicase
Abstract
Eukaryotic translation initiation is a highly regulated process in protein synthesis. The principal translation initiation factor eIF4AI displays helicase activity, unwinding secondary structures in the mRNAs 5'-UTR. Single molecule fluorescence resonance energy transfer (sm-FRET) is applied here to directly observe and quantify the helicase activity of eIF4AI in the presence of the ancillary RNA-binding factor eIF4H. Results show that eIF4H can significantly enhance the helicase activity of eIF4AI by strongly binding both to loop structures within the RNA transcript as well as to eIF4AI. In the presence of ATP, the eIF4AI/eIF4H complex exhibits persistent rapid and repetitive cycles of unwinding and re-annealing. ATP titration assays suggest that this process consumes a single ATP molecule per cycle. In contrast, helicase unwinding activity does not occur in the presence of the non-hydrolysable analog ATP-γS. Based on our sm-FRET results, we propose an unwinding mechanism where eIF4AI/eIF4H can bind directly to loop structures to destabilize duplexes. Since eIF4AI is the prototypical example of a DEA(D/H)-box RNA helicase, it is highly likely that this unwinding mechanism is applicable to a myriad of DEAD-box helicases employed in RNA metabolism.
Figures
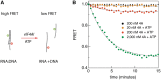
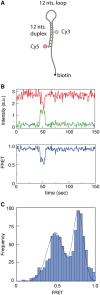
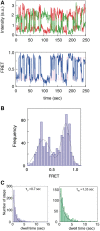





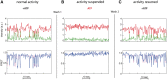
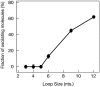
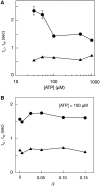





Similar articles
-
The DEAD-box helicase eIF4A: paradigm or the odd one out?RNA Biol. 2013 Jan;10(1):19-32. doi: 10.4161/rna.21966. Epub 2012 Sep 20. RNA Biol. 2013. PMID: 22995829 Free PMC article. Review.
-
Single-molecule kinetics of the eukaryotic initiation factor 4AI upon RNA unwinding.Structure. 2014 Jul 8;22(7):941-8. doi: 10.1016/j.str.2014.04.014. Epub 2014 Jun 5. Structure. 2014. PMID: 24909782
-
Interactions between eIF4AI and its accessory factors eIF4B and eIF4H.RNA. 2008 Oct;14(10):2136-48. doi: 10.1261/rna.1049608. Epub 2008 Aug 21. RNA. 2008. PMID: 18719248 Free PMC article.
-
Duplex unwinding and ATPase activities of the DEAD-box helicase eIF4A are coupled by eIF4G and eIF4B.J Mol Biol. 2011 Sep 30;412(4):674-87. doi: 10.1016/j.jmb.2011.08.004. Epub 2011 Aug 5. J Mol Biol. 2011. PMID: 21840318 Free PMC article.
-
Fluorescence methods in the investigation of the DEAD-box helicase mechanism.Exp Suppl. 2014;105:161-92. doi: 10.1007/978-3-0348-0856-9_8. Exp Suppl. 2014. PMID: 25095995 Review.
Cited by
-
The DEAD-box helicase eIF4A: paradigm or the odd one out?RNA Biol. 2013 Jan;10(1):19-32. doi: 10.4161/rna.21966. Epub 2012 Sep 20. RNA Biol. 2013. PMID: 22995829 Free PMC article. Review.
-
Translational Regulation by eIFs and RNA Modifications in Cancer.Genes (Basel). 2022 Nov 6;13(11):2050. doi: 10.3390/genes13112050. Genes (Basel). 2022. PMID: 36360287 Free PMC article. Review.
-
Toward a Kinetic Understanding of Eukaryotic Translation.Cold Spring Harb Perspect Biol. 2019 Feb 1;11(2):a032706. doi: 10.1101/cshperspect.a032706. Cold Spring Harb Perspect Biol. 2019. PMID: 29959192 Free PMC article. Review.
-
Risk model based on genes regulating the response of tumor cells to T-cell-mediated killing in esophageal squamous cell carcinoma.Aging (Albany NY). 2024 Feb 1;16(3):2494-2516. doi: 10.18632/aging.205495. Epub 2024 Feb 1. Aging (Albany NY). 2024. PMID: 38305770 Free PMC article.
-
Key contribution of eIF4H-mediated translational control in tumor promotion.Oncotarget. 2015 Nov 24;6(37):39924-40. doi: 10.18632/oncotarget.5442. Oncotarget. 2015. PMID: 26498689 Free PMC article.
References
-
- Sonenberg N. mRNA 5′ cap-binding protein elF4E and control of cell growth. In: Hershey JWB, Matthews MB, Sonenberg N, editors. Translational Control. Cold Spring Harbour, NY: Cold Spring Harbour Laboratory Press; 1996. pp. 245–269.
-
- Lamphear BJ, Kirchweger R, Skern T, Rhoads RE. Mapping of functional domains in eukaryotic protein synthesis initiation factor 4G (eIF4G) with picornaviral proteases. J. Biol. Chem. 1995;270:21975–21983. - PubMed
-
- Merrick WC, Hershey JWB. The pathway and mechanism of eukaryotic protein synthesis. In: Hershey JWB, Matthews BF, Sonenberg N, editors. Translational control. Cold Spring Harbor, NY: Cold Spring Harbor Laboratory Press; 1996. pp. 31–70.
-
- Pelletier J, Sonenberg N. Internal initiation of translation of eukaryotic messenger RNA directed by a sequence derived from poliovirus RNA. Nature. 1988;334:320–325. - PubMed
Publication types
MeSH terms
Substances
Grants and funding
LinkOut - more resources
Full Text Sources
Miscellaneous