A toolbox of Cre-dependent optogenetic transgenic mice for light-induced activation and silencing
- PMID: 22446880
- PMCID: PMC3337962
- DOI: 10.1038/nn.3078
A toolbox of Cre-dependent optogenetic transgenic mice for light-induced activation and silencing
Abstract
Cell type-specific expression of optogenetic molecules allows temporally precise manipulation of targeted neuronal activity. Here we present a toolbox of four knock-in mouse lines engineered for strong, Cre-dependent expression of channelrhodopsins ChR2-tdTomato and ChR2-EYFP, halorhodopsin eNpHR3.0 and archaerhodopsin Arch-ER2. All four transgenes mediated Cre-dependent, robust activation or silencing of cortical pyramidal neurons in vitro and in vivo upon light stimulation, with ChR2-EYFP and Arch-ER2 demonstrating light sensitivity approaching that of in utero or virally transduced neurons. We further show specific photoactivation of parvalbumin-positive interneurons in behaving ChR2-EYFP reporter mice. The robust, consistent and inducible nature of our ChR2 mice represents a significant advance over previous lines, and the Arch-ER2 and eNpHR3.0 mice are to our knowledge the first demonstration of successful conditional transgenic optogenetic silencing. When combined with the hundreds of available Cre driver lines, this optimized toolbox of reporter mice will enable widespread investigations of neural circuit function with unprecedented reliability and accuracy.
Figures
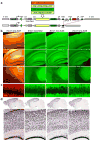
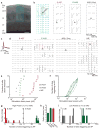
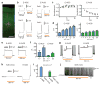
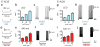
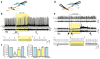
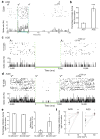
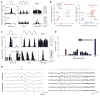
Similar articles
-
Light-induced silencing of neural activity in Rosa26 knock-in and BAC transgenic mice conditionally expressing the microbial halorhodopsin eNpHR3.Sci Rep. 2020 Feb 21;10(1):3191. doi: 10.1038/s41598-020-59984-3. Sci Rep. 2020. PMID: 32081938 Free PMC article.
-
Cell type–specific channelrhodopsin-2 transgenic mice for optogenetic dissection of neural circuitry function.Nat Methods. 2011 Sep;8(9):745-52. doi: 10.1038/nmeth.1668. Nat Methods. 2011. PMID: 21985008 Free PMC article.
-
Optogenetic approaches to characterize the long-range synaptic pathways from the hypothalamus to brain stem autonomic nuclei.J Neurosci Methods. 2012 Sep 30;210(2):238-46. doi: 10.1016/j.jneumeth.2012.07.022. Epub 2012 Aug 7. J Neurosci Methods. 2012. PMID: 22890236 Free PMC article.
-
Optogenetic tools for modulating and probing the epileptic network.Epilepsy Res. 2015 Oct;116:15-26. doi: 10.1016/j.eplepsyres.2015.06.010. Epub 2015 Jun 21. Epilepsy Res. 2015. PMID: 26354163 Free PMC article. Review.
-
WONOEP appraisal: optogenetic tools to suppress seizures and explore the mechanisms of epileptogenesis.Epilepsia. 2014 Nov;55(11):1693-702. doi: 10.1111/epi.12804. Epub 2014 Oct 9. Epilepsia. 2014. PMID: 25303540 Review.
Cited by
-
A deep learning approach to identifying immunogold particles in electron microscopy images.Sci Rep. 2021 Apr 8;11(1):7771. doi: 10.1038/s41598-021-87015-2. Sci Rep. 2021. PMID: 33833289 Free PMC article.
-
Contrast dependence and differential contributions from somatostatin- and parvalbumin-expressing neurons to spatial integration in mouse V1.J Neurosci. 2013 Jul 3;33(27):11145-54. doi: 10.1523/JNEUROSCI.5320-12.2013. J Neurosci. 2013. PMID: 23825418 Free PMC article.
-
Channelrhodopsin-assisted patching: in vivo recording of genetically and morphologically identified neurons throughout the brain.Cell Rep. 2014 Dec 24;9(6):2304-16. doi: 10.1016/j.celrep.2014.11.042. Epub 2014 Dec 18. Cell Rep. 2014. PMID: 25533350 Free PMC article.
-
Function and Circuitry of VIP+ Interneurons in the Mouse Retina.J Neurosci. 2015 Jul 29;35(30):10685-700. doi: 10.1523/JNEUROSCI.0222-15.2015. J Neurosci. 2015. PMID: 26224854 Free PMC article.
-
A valuable and promising method for recording brain activity in behaving newborn rodents.Dev Psychobiol. 2015 May;57(4):506-17. doi: 10.1002/dev.21305. Epub 2015 Apr 11. Dev Psychobiol. 2015. PMID: 25864710 Free PMC article.
References
-
- Boyden ES, Zhang F, Bamberg E, Nagel G, Deisseroth K. Millisecond-timescale, genetically targeted optical control of neural activity. Nat Neurosci. 2005;8:1263–1268. - PubMed
-
- Zhang F, Aravanis AM, Adamantidis A, de Lecea L, Deisseroth K. Circuit-breakers: optical technologies for probing neural signals and systems. Nat Rev Neurosci. 2007;8:577–581. - PubMed
Publication types
MeSH terms
Substances
Grants and funding
- MH54671/MH/NIMH NIH HHS/United States
- DA028298/DA/NIDA NIH HHS/United States
- MH093667/MH/NIMH NIH HHS/United States
- HHMI/Howard Hughes Medical Institute/United States
- MH90478/MH/NIMH NIH HHS/United States
- R01 MH054671/MH/NIMH NIH HHS/United States
- R01 NS034994/NS/NINDS NIH HHS/United States
- R21 MH090478/MH/NIMH NIH HHS/United States
- MH085944/MH/NIMH NIH HHS/United States
- NS034994/NS/NINDS NIH HHS/United States
- K99 MH085944/MH/NIMH NIH HHS/United States
- R01 DA028298/DA/NIDA NIH HHS/United States
- R01 DA028298-02/DA/NIDA NIH HHS/United States
- R01 MH093667/MH/NIMH NIH HHS/United States
- R01 HL107084/HL/NHLBI NIH HHS/United States
- R00 MH085944/MH/NIMH NIH HHS/United States
LinkOut - more resources
Full Text Sources
Other Literature Sources
Molecular Biology Databases
Research Materials