Cohesin-SA1 deficiency drives aneuploidy and tumourigenesis in mice due to impaired replication of telomeres
- PMID: 22415365
- PMCID: PMC3343459
- DOI: 10.1038/emboj.2012.11
Cohesin-SA1 deficiency drives aneuploidy and tumourigenesis in mice due to impaired replication of telomeres
Abstract
Cohesin is a protein complex originally identified for its role in sister chromatid cohesion, although increasing evidence portrays it also as a major organizer of interphase chromatin. Vertebrate cohesin consists of Smc1, Smc3, Rad21/Scc1 and either stromal antigen 1 (SA1) or SA2. To explore the functional specificity of these two versions of cohesin and their relevance for embryonic development and cancer, we generated a mouse model deficient for SA1. Complete ablation of SA1 results in embryonic lethality, while heterozygous animals have shorter lifespan and earlier onset of tumourigenesis. SA1-null mouse embryonic fibroblasts show decreased proliferation and increased aneuploidy as a result of chromosome segregation defects. These defects are not caused by impaired centromeric cohesion, which depends on cohesin-SA2. Instead, they arise from defective telomere replication, which requires cohesion mediated specifically by cohesin-SA1. We propose a novel mechanism for aneuploidy generation that involves impaired telomere replication upon loss of cohesin-SA1, with clear implications in tumourigenesis.
Conflict of interest statement
The authors declare that they have no conflict of interest.
Figures
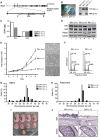
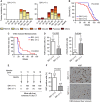
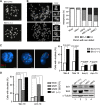
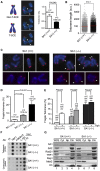
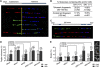
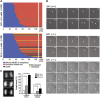
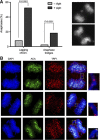
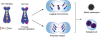
Comment in
-
The many functions of cohesin--different rings to rule them all?EMBO J. 2012 May 2;31(9):2061-3. doi: 10.1038/emboj.2012.90. Epub 2012 Apr 10. EMBO J. 2012. PMID: 22491011 Free PMC article.
Similar articles
-
The specific contributions of cohesin-SA1 to cohesion and gene expression: implications for cancer and development.Cell Cycle. 2012 Jun 15;11(12):2233-8. doi: 10.4161/cc.20318. Epub 2012 Jun 15. Cell Cycle. 2012. PMID: 22617390
-
A unique role of cohesin-SA1 in gene regulation and development.EMBO J. 2012 May 2;31(9):2090-102. doi: 10.1038/emboj.2012.60. Epub 2012 Mar 13. EMBO J. 2012. PMID: 22415368 Free PMC article.
-
Characterization of the interaction between the cohesin subunits Rad21 and SA1/2.PLoS One. 2013 Jul 12;8(7):e69458. doi: 10.1371/journal.pone.0069458. Print 2013. PLoS One. 2013. PMID: 23874961 Free PMC article.
-
The expanding phenotypes of cohesinopathies: one ring to rule them all!Cell Cycle. 2019 Nov;18(21):2828-2848. doi: 10.1080/15384101.2019.1658476. Epub 2019 Sep 13. Cell Cycle. 2019. PMID: 31516082 Free PMC article. Review.
-
Functional Coupling between DNA Replication and Sister Chromatid Cohesion Establishment.Int J Mol Sci. 2021 Mar 10;22(6):2810. doi: 10.3390/ijms22062810. Int J Mol Sci. 2021. PMID: 33802105 Free PMC article. Review.
Cited by
-
SA1 binds directly to DNA through its unique AT-hook to promote sister chromatid cohesion at telomeres.J Cell Sci. 2013 Aug 1;126(Pt 15):3493-503. doi: 10.1242/jcs.130872. Epub 2013 May 31. J Cell Sci. 2013. PMID: 23729739 Free PMC article.
-
Diverse developmental disorders from the one ring: distinct molecular pathways underlie the cohesinopathies.Front Genet. 2012 Sep 12;3:171. doi: 10.3389/fgene.2012.00171. eCollection 2012. Front Genet. 2012. PMID: 22988450 Free PMC article.
-
PCNA antagonizes cohesin-dependent roles in genomic stability.PLoS One. 2020 Oct 19;15(10):e0235103. doi: 10.1371/journal.pone.0235103. eCollection 2020. PLoS One. 2020. PMID: 33075068 Free PMC article.
-
Matrin-3 acts as a potential biomarker and promotes hepatocellular carcinoma progression by interacting with cell cycle-regulating genes.Cell Cycle. 2024 Jan;23(1):15-35. doi: 10.1080/15384101.2024.2305535. Epub 2024 Jan 22. Cell Cycle. 2024. PMID: 38252499 Free PMC article.
-
STAG1 vulnerabilities for exploiting cohesin synthetic lethality in STAG2-deficient cancers.Life Sci Alliance. 2020 May 28;3(7):e202000725. doi: 10.26508/lsa.202000725. Print 2020 Jul. Life Sci Alliance. 2020. PMID: 32467316 Free PMC article.
References
Publication types
MeSH terms
Substances
Grants and funding
LinkOut - more resources
Full Text Sources
Molecular Biology Databases
Miscellaneous