Distinct neuronal coding schemes in memory revealed by selective erasure of fast synchronous synaptic transmission
- PMID: 22405208
- PMCID: PMC3319466
- DOI: 10.1016/j.neuron.2011.12.036
Distinct neuronal coding schemes in memory revealed by selective erasure of fast synchronous synaptic transmission
Abstract
Neurons encode information by firing spikes in isolation or bursts and propagate information by spike-triggered neurotransmitter release that initiates synaptic transmission. Isolated spikes trigger neurotransmitter release unreliably but with high temporal precision. In contrast, bursts of spikes trigger neurotransmission reliably (i.e., boost transmission fidelity), but the resulting synaptic responses are temporally imprecise. However, the relative physiological importance of different spike-firing modes remains unclear. Here, we show that knockdown of synaptotagmin-1, the major Ca(2+) sensor for neurotransmitter release, abrogated neurotransmission evoked by isolated spikes but only delayed, without abolishing, neurotransmission evoked by bursts of spikes. Nevertheless, knockdown of synaptotagmin-1 in the hippocampal CA1 region did not impede acquisition of recent contextual fear memories, although it did impair the precision of such memories. In contrast, knockdown of synaptotagmin-1 in the prefrontal cortex impaired all remote fear memories. These results indicate that different brain circuits and types of memory employ distinct spike-coding schemes to encode and transmit information.
Copyright © 2012 Elsevier Inc. All rights reserved.
Figures
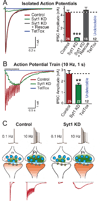
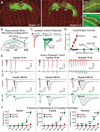
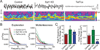
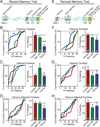
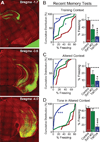
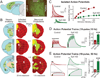
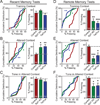
Comment in
-
How do neurons sense a spike burst?Neuron. 2012 Mar 8;73(5):857-9. doi: 10.1016/j.neuron.2012.02.013. Neuron. 2012. PMID: 22405197
Similar articles
-
Dynamic balance of excitation and inhibition rapidly modulates spike probability and precision in feed-forward hippocampal circuits.J Neurophysiol. 2016 Dec 1;116(6):2564-2575. doi: 10.1152/jn.00413.2016. Epub 2016 Sep 7. J Neurophysiol. 2016. PMID: 27605532 Free PMC article.
-
Synaptic transmission changes in fear memory circuits underlie key features of an animal model of schizophrenia.Behav Brain Res. 2012 Feb 1;227(1):184-93. doi: 10.1016/j.bbr.2011.10.050. Epub 2011 Nov 9. Behav Brain Res. 2012. PMID: 22085880
-
Limbic gamma rhythms. II. Synaptic and intrinsic mechanisms underlying spike doublets in oscillating subicular neurons.J Neurophysiol. 1998 Jul;80(1):162-71. doi: 10.1152/jn.1998.80.1.162. J Neurophysiol. 1998. PMID: 9658038
-
Autapses and networks of hippocampal neurons exhibit distinct synaptic transmission phenotypes in the absence of synaptotagmin I.J Neurosci. 2009 Jun 10;29(23):7395-403. doi: 10.1523/JNEUROSCI.1341-09.2009. J Neurosci. 2009. PMID: 19515907 Free PMC article.
-
Vesicle dynamics: how synaptic proteins regulate different modes of neurotransmission.J Neurochem. 2013 Jul;126(2):146-54. doi: 10.1111/jnc.12245. Epub 2013 Apr 23. J Neurochem. 2013. PMID: 23517499 Review.
Cited by
-
Neuromodulator release in neurons requires two functionally redundant calcium sensors.Proc Natl Acad Sci U S A. 2021 May 4;118(18):e2012137118. doi: 10.1073/pnas.2012137118. Proc Natl Acad Sci U S A. 2021. PMID: 33903230 Free PMC article.
-
Cocaine-Induced Synaptic Alterations in Thalamus to Nucleus Accumbens Projection.Neuropsychopharmacology. 2016 Aug;41(9):2399-410. doi: 10.1038/npp.2016.52. Epub 2016 Apr 14. Neuropsychopharmacology. 2016. PMID: 27074816 Free PMC article.
-
Synaptotagmin-1 is a Ca2+ sensor for somatodendritic dopamine release.Cell Rep. 2023 Jan 31;42(1):111915. doi: 10.1016/j.celrep.2022.111915. Epub 2022 Dec 30. Cell Rep. 2023. PMID: 36640316 Free PMC article.
-
Autism-associated SHANK3 haploinsufficiency causes Ih channelopathy in human neurons.Science. 2016 May 6;352(6286):aaf2669. doi: 10.1126/science.aaf2669. Epub 2016 Mar 10. Science. 2016. PMID: 26966193 Free PMC article.
-
Modulation of excitation on parvalbumin interneurons by neuroligin-3 regulates the hippocampal network.Nat Neurosci. 2017 Feb;20(2):219-229. doi: 10.1038/nn.4471. Epub 2017 Jan 9. Nat Neurosci. 2017. PMID: 28067903 Free PMC article.
References
Publication types
MeSH terms
Substances
Grants and funding
LinkOut - more resources
Full Text Sources
Other Literature Sources
Medical
Molecular Biology Databases
Miscellaneous