Phosphorylation by protein kinase Cα regulates RalB small GTPase protein activation, subcellular localization, and effector utilization
- PMID: 22393054
- PMCID: PMC3340265
- DOI: 10.1074/jbc.M112.344986
Phosphorylation by protein kinase Cα regulates RalB small GTPase protein activation, subcellular localization, and effector utilization
Abstract
Ras-like (Ral) small GTPases are regulated downstream of Ras and the noncanonical Ral guanine nucleotide exchange factor (RalGEF) effector pathway. Despite RalA and RalB sharing 82% sequence identity and utilization of shared effector proteins, their roles in normal and neoplastic cell growth have been shown to be highly distinct. Here, we determined that RalB function is regulated by protein kinase Cα (PKCα) phosphorylation. We found that RalB phosphorylation on Ser-198 in the C-terminal membrane targeting sequence resulted in enhanced RalB endomembrane accumulation and decreased RalB association with its effector, the exocyst component Sec5. Additionally, RalB phosphorylation regulated vesicular trafficking and membrane fusion by regulating v- and t-SNARE interactions. RalB phosphorylation regulated vesicular traffic of α5-integrin to the cell surface and cell attachment to fibronectin. In summary, our data suggest that phosphorylation by PKCα is critical for RalB-mediated vesicle trafficking and exocytosis.
Figures
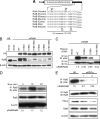
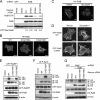
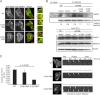
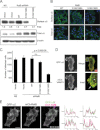
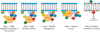
Similar articles
-
The RAL Enigma: Distinct Roles of RALA and RALB in Cancer.Cells. 2022 May 14;11(10):1645. doi: 10.3390/cells11101645. Cells. 2022. PMID: 35626682 Free PMC article. Review.
-
Divergent roles of CAAX motif-signaled posttranslational modifications in the regulation and subcellular localization of Ral GTPases.J Biol Chem. 2015 Sep 11;290(37):22851-61. doi: 10.1074/jbc.M115.656710. Epub 2015 Jul 27. J Biol Chem. 2015. PMID: 26216878 Free PMC article.
-
Solution structure and dynamics of the small GTPase RalB in its active conformation: significance for effector protein binding.Biochemistry. 2009 Mar 17;48(10):2192-206. doi: 10.1021/bi802129d. Biochemistry. 2009. PMID: 19166349
-
Distinct roles of RalA and RalB in the progression of cytokinesis are supported by distinct RalGEFs.EMBO J. 2008 Sep 17;27(18):2375-87. doi: 10.1038/emboj.2008.166. Epub 2008 Aug 28. EMBO J. 2008. PMID: 18756269 Free PMC article.
-
The RAL signaling network: Cancer and beyond.Int Rev Cell Mol Biol. 2021;361:21-105. doi: 10.1016/bs.ircmb.2020.10.005. Epub 2020 Dec 2. Int Rev Cell Mol Biol. 2021. PMID: 34074494 Review.
Cited by
-
The RAL Enigma: Distinct Roles of RALA and RALB in Cancer.Cells. 2022 May 14;11(10):1645. doi: 10.3390/cells11101645. Cells. 2022. PMID: 35626682 Free PMC article. Review.
-
RabGAP22 is required for defense to the vascular pathogen Verticillium longisporum and contributes to stomata immunity.PLoS One. 2014 Feb 4;9(2):e88187. doi: 10.1371/journal.pone.0088187. eCollection 2014. PLoS One. 2014. PMID: 24505423 Free PMC article.
-
Drugging the Ral GTPase.Small GTPases. 2015;6(3):157-9. doi: 10.1080/21541248.2015.1018403. Small GTPases. 2015. PMID: 26280620 Free PMC article.
-
Ral GTPase down-regulation stabilizes and reactivates p53 to inhibit malignant transformation.J Biol Chem. 2014 Nov 7;289(45):31296-309. doi: 10.1074/jbc.M114.565796. Epub 2014 Sep 10. J Biol Chem. 2014. PMID: 25210032 Free PMC article.
-
RalB uncoupled exocyst mediates endothelial Weibel-Palade body exocytosis.bioRxiv [Preprint]. 2024 Sep 16:2024.09.16.613344. doi: 10.1101/2024.09.16.613344. bioRxiv. 2024. PMID: 39345530 Free PMC article. Preprint.
References
-
- Bodemann B. O., White M. A. (2008) Ral GTPases and cancer. Linchpin support of the tumorigenic platform. Nat. Rev. Cancer 8, 133–140 - PubMed
-
- Lim K. H., O'Hayer K., Adam S. J., Kendall S. D., Campbell P. M., Der C. J., Counter C. M. (2006) Divergent roles for RalA and RalB in malignant growth of human pancreatic carcinoma cells. Curr. Biol. 16, 2385–2394 - PubMed
-
- Lim K. H., Baines A. T., Fiordalisi J. J., Shipitsin M., Feig L. A., Cox A. D., Der C. J., Counter C. M. (2005) Activation of RalA is critical for Ras-induced tumorigenesis of human cells. Cancer Cell 7, 533–545 - PubMed
Publication types
MeSH terms
Substances
Grants and funding
LinkOut - more resources
Full Text Sources
Molecular Biology Databases
Research Materials
Miscellaneous