Photochemical mechanisms of light-triggered release from nanocarriers
- PMID: 22386560
- PMCID: PMC3395781
- DOI: 10.1016/j.addr.2012.02.006
Photochemical mechanisms of light-triggered release from nanocarriers
Abstract
Over the last three decades, a handful of photochemical mechanisms have been applied to a large number of nanoscale assemblies that encapsulate a payload to afford spatio-temporal and remote control over activity of the encapsulated payload. Many of these systems are designed with an eye towards biomedical applications, as spatio-temporal and remote control of bioactivity would advance research and clinical practice. This review covers five underlying photochemical mechanisms that govern the activity of the majority of photoresponsive nanocarriers: 1. photo driven isomerization and oxidation, 2. surface plasmon absorption and photothermal effects, 3. photo driven hydrophobicity changes, 4. photo driven polymer backbone fragmentation and 5. photo driven de-crosslinking. The ways in which these mechanisms have been incorporated into nanocarriers and how they affect release are detailed, as well as the advantages and disadvantages of each system.
Copyright © 2012 Elsevier B.V. All rights reserved.
Figures
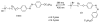
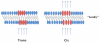
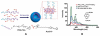
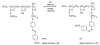
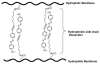
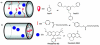
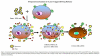
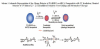
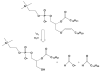
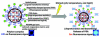
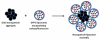
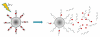
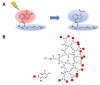
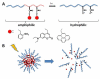
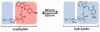
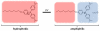
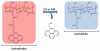
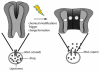
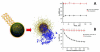
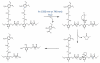
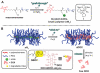
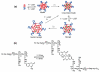
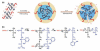
Similar articles
-
Photoresponsive hydrogels for biomedical applications.Adv Drug Deliv Rev. 2011 Nov;63(14-15):1257-66. doi: 10.1016/j.addr.2011.06.009. Epub 2011 Jul 2. Adv Drug Deliv Rev. 2011. PMID: 21745509 Review.
-
Smart Nanostructures for Cargo Delivery: Uncaging and Activating by Light.J Am Chem Soc. 2017 Apr 5;139(13):4584-4610. doi: 10.1021/jacs.6b08313. Epub 2017 Mar 13. J Am Chem Soc. 2017. PMID: 28192672 Free PMC article. Review.
-
Photo-responsive polymeric nanocarriers for target-specific and controlled drug delivery.Soft Matter. 2021 Oct 6;17(38):8577-8584. doi: 10.1039/d1sm00999k. Soft Matter. 2021. PMID: 34580698 Review.
-
Recent advances in near-infrared light-responsive nanocarriers for cancer therapy.Drug Discov Today. 2018 May;23(5):1115-1125. doi: 10.1016/j.drudis.2018.02.005. Epub 2018 Feb 23. Drug Discov Today. 2018. PMID: 29481876 Review.
-
Photoresponsive crosslinked hyperbranched polyglycerols as smart nanocarriers for guest binding and controlled release.Small. 2009 Oct;5(19):2199-204. doi: 10.1002/smll.200900465. Small. 2009. PMID: 19572327
Cited by
-
Fabrication and Characterisation of a Photo-Responsive, Injectable Nanosystem for Sustained Delivery of Macromolecules.Int J Mol Sci. 2021 Mar 25;22(7):3359. doi: 10.3390/ijms22073359. Int J Mol Sci. 2021. PMID: 33805969 Free PMC article.
-
Nanomaterials for photo-based diagnostic and therapeutic applications.Theranostics. 2013;3(3):152-66. doi: 10.7150/thno.5327. Epub 2013 Feb 20. Theranostics. 2013. PMID: 23471164 Free PMC article. Review.
-
Biotransporting Biocatalytic Reactors toward Therapeutic Nanofactories.Adv Sci (Weinh). 2018 Sep 19;5(11):1800801. doi: 10.1002/advs.201800801. eCollection 2018 Nov. Adv Sci (Weinh). 2018. PMID: 30479925 Free PMC article.
-
NIR-driven Smart Theranostic Nanomedicine for On-demand Drug Release and Synergistic Antitumour Therapy.Sci Rep. 2015 Sep 24;5:14258. doi: 10.1038/srep14258. Sci Rep. 2015. PMID: 26400780 Free PMC article.
-
Recent advances in light-responsive on-demand drug-delivery systems.Ther Deliv. 2017 Feb;8(2):89-107. doi: 10.4155/tde-2016-0060. Ther Deliv. 2017. PMID: 28088880 Free PMC article. Review.
References
-
- Weissleder R. A clearer vision for in vivo imaging. Nat. Biotechnol. 2001;19:316–317. - PubMed
-
- Shum P, Kim J-M, Thompson DH. Phototriggering of liposomal drug delivery systems. Adv. Drug Del. Rev. 2001;53:273–284. - PubMed
-
- Alvarez-Lorenzo C, Bromberg L, Concheiro A. Light-sensitive intelligent drug delivery systems. Photochem. Photobiol. 2009;85:848–860. - PubMed
-
- Zhao Y. Photocontrollable block copolymer micelles: what can we control? J. Mater. Chem. 2009;19:4887–4895.
Publication types
MeSH terms
Substances
Grants and funding
LinkOut - more resources
Full Text Sources
Other Literature Sources