Rational design of small molecule inhibitors targeting the Rac GTPase-p67(phox) signaling axis in inflammation
- PMID: 22365606
- PMCID: PMC3292765
- DOI: 10.1016/j.chembiol.2011.12.017
Rational design of small molecule inhibitors targeting the Rac GTPase-p67(phox) signaling axis in inflammation
Abstract
The NADPH oxidase enzyme complex, NOX2, is responsible for reactive oxygen species production in neutrophils and has been recognized as a key mediator of inflammation. Here, we have performed rational design and in silico screen to identify a small molecule inhibitor, Phox-I1, targeting the interactive site of p67(phox) with Rac GTPase, which is a necessary step of the signaling leading to NOX2 activation. Phox-I1 binds to p67(phox) with a submicromolar affinity and abrogates Rac1 binding and is effective in inhibiting NOX2-mediated superoxide production dose-dependently in human and murine neutrophils without detectable toxicity. Medicinal chemistry characterizations have yielded promising analogs and initial information of the structure-activity relationship of Phox-I1. Our studies suggest the potential utility of Phox-I class inhibitors in NOX2 oxidase inhibition and present an application of rational targeting of a small GTPase-effector interface.
Copyright © 2012 Elsevier Ltd. All rights reserved.
Conflict of interest statement
The authors have no conflict of interest to declare.
Figures
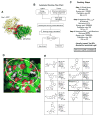
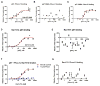
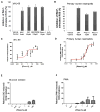
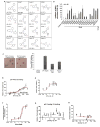
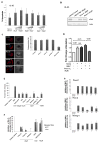
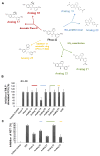
Comment in
-
Exploiting effectors of Rac GTPase.Chem Biol. 2012 Feb 24;19(2):169-71. doi: 10.1016/j.chembiol.2012.02.001. Chem Biol. 2012. PMID: 22365599 Free PMC article.
Similar articles
-
Small molecule targeting the Rac1-NOX2 interaction prevents collagen-related peptide and thrombin-induced reactive oxygen species generation and platelet activation.J Thromb Haemost. 2018 Oct;16(10):2083-2096. doi: 10.1111/jth.14240. Epub 2018 Aug 13. J Thromb Haemost. 2018. PMID: 30007118 Free PMC article.
-
Exploiting effectors of Rac GTPase.Chem Biol. 2012 Feb 24;19(2):169-71. doi: 10.1016/j.chembiol.2012.02.001. Chem Biol. 2012. PMID: 22365599 Free PMC article.
-
The molecular basis of Rac-GTP action-promoting binding of p67phox to Nox2 by disengaging the β hairpin from downstream residues.J Leukoc Biol. 2021 Aug;110(2):219-237. doi: 10.1002/JLB.4HI1220-855RR. Epub 2021 Apr 15. J Leukoc Biol. 2021. PMID: 33857329
-
Role of the small GTPase Rac in p22phox-dependent NADPH oxidases.Biochimie. 2007 Sep;89(9):1133-44. doi: 10.1016/j.biochi.2007.05.003. Epub 2007 May 17. Biochimie. 2007. PMID: 17583407 Review.
-
Arachidonic Acid and Nitroarachidonic: Effects on NADPH Oxidase Activity.Adv Exp Med Biol. 2019;1127:85-95. doi: 10.1007/978-3-030-11488-6_6. Adv Exp Med Biol. 2019. PMID: 31140173 Review.
Cited by
-
The novel NADPH oxidase 4 selective inhibitor GLX7013114 counteracts human islet cell death in vitro.PLoS One. 2018 Sep 28;13(9):e0204271. doi: 10.1371/journal.pone.0204271. eCollection 2018. PLoS One. 2018. PMID: 30265686 Free PMC article.
-
Small molecule targeting the Rac1-NOX2 interaction prevents collagen-related peptide and thrombin-induced reactive oxygen species generation and platelet activation.J Thromb Haemost. 2018 Oct;16(10):2083-2096. doi: 10.1111/jth.14240. Epub 2018 Aug 13. J Thromb Haemost. 2018. PMID: 30007118 Free PMC article.
-
NADPH oxidase family proteins: signaling dynamics to disease management.Cell Mol Immunol. 2022 Jun;19(6):660-686. doi: 10.1038/s41423-022-00858-1. Epub 2022 May 18. Cell Mol Immunol. 2022. PMID: 35585127 Free PMC article. Review.
-
Phagocyte-like NADPH oxidase [Nox2] in cellular dysfunction in models of glucolipotoxicity and diabetes.Biochem Pharmacol. 2014 Apr 1;88(3):275-83. doi: 10.1016/j.bcp.2014.01.017. Epub 2014 Jan 22. Biochem Pharmacol. 2014. PMID: 24462914 Free PMC article.
-
Modeling host genetic regulation of influenza pathogenesis in the collaborative cross.PLoS Pathog. 2013 Feb;9(2):e1003196. doi: 10.1371/journal.ppat.1003196. Epub 2013 Feb 28. PLoS Pathog. 2013. PMID: 23468633 Free PMC article.
References
-
- Abo A, Pick E, Hall A, Totty N, Teahan CG, Segal AW. Activation of the NADPH oxidase involves the small GTP-binding protein p21rac1. Nature. 1991;353:668–670. - PubMed
-
- Ahmed S, Prigmore E, Govind S, Veryard C, Kozma R, Wientjes FB, Segal AW, Lim L. Cryptic Rac-binding and p21(Cdc42Hs/Rac)-activated kinase phosphorylation sites of NADPH oxidase component p67(phox) J Biol Chem. 1998;273:15693–15701. - PubMed
-
- Aldieri E, Riganti C, Polimeni M, Gazzano E, Lussiana C, Campia I, Ghigo D. Classical inhibitors of NOX NAD(P)H oxidases are not specific. Curr Drug Metab. 2008;9:686–696. - PubMed
-
- Bedard K, Krause KH. The NOX family of ROS-generating NADPH oxidases: physiology and pathophysiology. Physiol Rev. 2007;87:245–313. - PubMed
Publication types
MeSH terms
Substances
Grants and funding
LinkOut - more resources
Full Text Sources
Other Literature Sources
Research Materials
Miscellaneous