A mammary stem cell population identified and characterized in late embryogenesis reveals similarities to human breast cancer
- PMID: 22305568
- PMCID: PMC3277444
- DOI: 10.1016/j.stem.2011.12.018
A mammary stem cell population identified and characterized in late embryogenesis reveals similarities to human breast cancer
Abstract
Gene expression signatures relating mammary stem cell populations to breast cancers have focused on adult tissue. Here, we identify, isolate, and characterize the fetal mammary stem cell (fMaSC) state since the invasive and proliferative processes of mammogenesis resemble phases of cancer progression. fMaSC frequency peaks late in embryogenesis, enabling more extensive stem cell purification than achieved with adult tissue. fMaSCs are self-renewing, multipotent, and coexpress multiple mammary lineage markers. Gene expression, transplantation, and in vitro analyses reveal putative autocrine and paracrine regulatory mechanisms, including ErbB and FGF signaling pathways impinging on fMaSC growth. Expression profiles from fMaSCs and associated stroma exhibit significant similarities to basal-like and Her2+ intrinsic breast cancer subtypes. Our results reveal links between development and cancer and provide resources to identify new candidates for diagnosis, prognosis, and therapy.
Copyright © 2012 Elsevier Inc. All rights reserved.
Figures
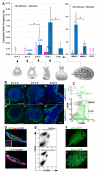
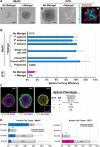
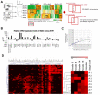
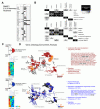
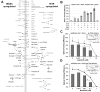
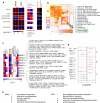
Similar articles
-
Mammary development and breast cancer: the role of stem cells.Curr Mol Med. 2011 Jun;11(4):270-85. doi: 10.2174/156652411795678007. Curr Mol Med. 2011. PMID: 21506923 Free PMC article. Review.
-
Characterization of cell lines derived from breast cancers and normal mammary tissues for the study of the intrinsic molecular subtypes.Breast Cancer Res Treat. 2013 Nov;142(2):237-55. doi: 10.1007/s10549-013-2743-3. Epub 2013 Oct 27. Breast Cancer Res Treat. 2013. PMID: 24162158 Free PMC article.
-
Normal and cancerous mammary stem cells evade interferon-induced constraint through the miR-199a-LCOR axis.Nat Cell Biol. 2017 Jun;19(6):711-723. doi: 10.1038/ncb3533. Epub 2017 May 22. Nat Cell Biol. 2017. PMID: 28530657 Free PMC article.
-
Developmental signaling pathways regulating mammary stem cells and contributing to the etiology of triple-negative breast cancer.Breast Cancer Res Treat. 2016 Apr;156(2):211-26. doi: 10.1007/s10549-016-3746-7. Epub 2016 Mar 11. Breast Cancer Res Treat. 2016. PMID: 26968398 Free PMC article. Review.
-
Sox10 Regulates Stem/Progenitor and Mesenchymal Cell States in Mammary Epithelial Cells.Cell Rep. 2015 Sep 29;12(12):2035-48. doi: 10.1016/j.celrep.2015.08.040. Epub 2015 Sep 10. Cell Rep. 2015. PMID: 26365194 Free PMC article.
Cited by
-
Development of Foreign Mammary Epithelial Morphology in the Stroma of Immunodeficient Mice.PLoS One. 2013 Jun 18;8(6):e68637. doi: 10.1371/journal.pone.0068637. Print 2013. PLoS One. 2013. PMID: 23825700 Free PMC article.
-
The Cellular Organization of the Mammary Gland: Insights From Microscopy.J Mammary Gland Biol Neoplasia. 2021 Mar;26(1):71-85. doi: 10.1007/s10911-021-09483-6. Epub 2021 Apr 9. J Mammary Gland Biol Neoplasia. 2021. PMID: 33835387 Review.
-
Lgr5-expressing cells are sufficient and necessary for postnatal mammary gland organogenesis.Cell Rep. 2013 Jan 31;3(1):70-8. doi: 10.1016/j.celrep.2012.12.017. Epub 2013 Jan 24. Cell Rep. 2013. PMID: 23352663 Free PMC article.
-
AXL Is a Driver of Stemness in Normal Mammary Gland and Breast Cancer.iScience. 2020 Oct 7;23(11):101649. doi: 10.1016/j.isci.2020.101649. eCollection 2020 Nov 20. iScience. 2020. PMID: 33103086 Free PMC article.
-
Cell Reprogramming in Tumorigenesis and Its Therapeutic Implications for Breast Cancer.Int J Mol Sci. 2019 Apr 12;20(8):1827. doi: 10.3390/ijms20081827. Int J Mol Sci. 2019. PMID: 31013830 Free PMC article. Review.
References
-
- Anbazhagan R, Osin PP, Bartkova J, Nathan B, Lane EB, Gusterson BA. The development of epithelial phenotypes in the human fetal and infant breast. J Pathol. 1998;184:197–206. - PubMed
-
- Bonnefoix T, Bonnefoix P, Verdiel P, Sotto JJ. Fitting limiting dilution experiments with generalized linear models results in a test of the single-hit Poisson assumption. J Immunol Methods. 1996;194:113–119. - PubMed
-
- Brewer BG, Mitchell RA, Harandi A, Eaton JW. Embryonic vaccines against cancer: an early history. Exp Mol Pathol. 2009;86:192–197. - PubMed
Publication types
MeSH terms
Substances
Associated data
- Actions
Grants and funding
LinkOut - more resources
Full Text Sources
Other Literature Sources
Medical
Molecular Biology Databases
Research Materials
Miscellaneous