TRPA1 channels regulate astrocyte resting calcium and inhibitory synapse efficacy through GAT-3
- PMID: 22158513
- PMCID: PMC3282183
- DOI: 10.1038/nn.3000
TRPA1 channels regulate astrocyte resting calcium and inhibitory synapse efficacy through GAT-3
Abstract
Astrocytes contribute to the formation and function of synapses and are found throughout the brain, where they show intracellular store-mediated Ca(2+) signals. Here, using a membrane-tethered, genetically encoded calcium indicator (Lck-GCaMP3), we report the serendipitous discovery of a new type of Ca(2+) signal in rat hippocampal astrocyte-neuron cocultures. We found that Ca(2+) fluxes mediated by transient receptor potential A1 (TRPA1) channels gave rise to frequent and highly localized 'spotty' Ca(2+) microdomains near the membrane that contributed appreciably to resting Ca(2+) in astrocytes. Mechanistic evaluations in brain slices showed that decreases in astrocyte resting Ca(2+) concentrations mediated by TRPA1 channels decreased interneuron inhibitory synapse efficacy by reducing GABA transport by GAT-3, thus elevating extracellular GABA. Our data show how a transmembrane Ca(2+) source (TRPA1) targets a transporter (GAT-3) in astrocytes to regulate inhibitory synapses.
Figures
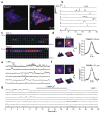
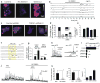
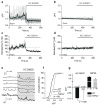
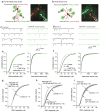
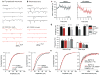
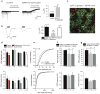
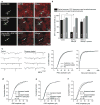
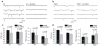
Comment in
-
An astrocyte TRP switch for inhibition.Nat Neurosci. 2011 Dec 23;15(1):3-4. doi: 10.1038/nn.3010. Nat Neurosci. 2011. PMID: 22193248 No abstract available.
Similar articles
-
Astrocyte Ca2+ Influx Negatively Regulates Neuronal Activity.eNeuro. 2017 Mar 10;4(2):ENEURO.0340-16.2017. doi: 10.1523/ENEURO.0340-16.2017. eCollection 2017 Mar-Apr. eNeuro. 2017. PMID: 28303263 Free PMC article.
-
An astrocyte TRP switch for inhibition.Nat Neurosci. 2011 Dec 23;15(1):3-4. doi: 10.1038/nn.3010. Nat Neurosci. 2011. PMID: 22193248 No abstract available.
-
Genetically encoded calcium indicators and astrocyte calcium microdomains.Neuroscientist. 2013 Jun;19(3):274-91. doi: 10.1177/1073858412468794. Epub 2012 Dec 20. Neuroscientist. 2013. PMID: 23264008
-
Local energy on demand: Are 'spontaneous' astrocytic Ca2+-microdomains the regulatory unit for astrocyte-neuron metabolic cooperation?Brain Res Bull. 2018 Jan;136:54-64. doi: 10.1016/j.brainresbull.2017.04.011. Epub 2017 Apr 24. Brain Res Bull. 2018. PMID: 28450076 Review.
-
TRP channels coordinate ion signalling in astroglia.Rev Physiol Biochem Pharmacol. 2014;166:1-22. doi: 10.1007/112_2013_15. Rev Physiol Biochem Pharmacol. 2014. PMID: 23784619 Free PMC article. Review.
Cited by
-
Inflammatory mediators alter the astrocyte transcriptome and calcium signaling elicited by multiple G-protein-coupled receptors.J Neurosci. 2012 Oct 17;32(42):14489-510. doi: 10.1523/JNEUROSCI.1256-12.2012. J Neurosci. 2012. PMID: 23077035 Free PMC article.
-
Mapping brain gene coexpression in daytime transcriptomes unveils diurnal molecular networks and deciphers perturbation gene signatures.Neuron. 2022 Oct 19;110(20):3318-3338.e9. doi: 10.1016/j.neuron.2022.09.028. Neuron. 2022. PMID: 36265442 Free PMC article.
-
An Astrocytic Influence on Impaired Tonic Inhibition in Hippocampal CA1 Pyramidal Neurons in a Mouse Model of Rett Syndrome.J Neurosci. 2020 Aug 5;40(32):6250-6261. doi: 10.1523/JNEUROSCI.3042-19.2020. Epub 2020 Jul 2. J Neurosci. 2020. PMID: 32616668 Free PMC article.
-
Diversity of astrocyte functions and phenotypes in neural circuits.Nat Neurosci. 2015 Jul;18(7):942-52. doi: 10.1038/nn.4043. Nat Neurosci. 2015. PMID: 26108722 Free PMC article. Review.
-
Hydrogen sulfide and polysulfides as signaling molecules.Proc Jpn Acad Ser B Phys Biol Sci. 2015;91(4):131-59. doi: 10.2183/pjab.91.131. Proc Jpn Acad Ser B Phys Biol Sci. 2015. PMID: 25864468 Free PMC article. Review.
References
Publication types
MeSH terms
Substances
Grants and funding
- R21 NS063186-01/NS/NINDS NIH HHS/United States
- R21 NS071292-02/NS/NINDS NIH HHS/United States
- R21 NS071292/NS/NINDS NIH HHS/United States
- NS060677/NS/NINDS NIH HHS/United States
- R01 NS060677/NS/NINDS NIH HHS/United States
- R01 NS060677-03/NS/NINDS NIH HHS/United States
- R21 NS063186/NS/NINDS NIH HHS/United States
- NS071292/NS/NINDS NIH HHS/United States
- R01 NS060677-04/NS/NINDS NIH HHS/United States
- R01 DC000304/DC/NIDCD NIH HHS/United States
- R01 NS060677-02/NS/NINDS NIH HHS/United States
- R21 NS071292-01/NS/NINDS NIH HHS/United States
- R21 NS063186-02S1/NS/NINDS NIH HHS/United States
- R01 NS060677-01A1/NS/NINDS NIH HHS/United States
- NS063186/NS/NINDS NIH HHS/United States
LinkOut - more resources
Full Text Sources
Other Literature Sources
Molecular Biology Databases
Miscellaneous