Selective ribosome profiling reveals the cotranslational chaperone action of trigger factor in vivo
- PMID: 22153074
- PMCID: PMC3277850
- DOI: 10.1016/j.cell.2011.10.044
Selective ribosome profiling reveals the cotranslational chaperone action of trigger factor in vivo
Abstract
As nascent polypeptides exit ribosomes, they are engaged by a series of processing, targeting, and folding factors. Here, we present a selective ribosome profiling strategy that enables global monitoring of when these factors engage polypeptides in the complex cellular environment. Studies of the Escherichia coli chaperone trigger factor (TF) reveal that, though TF can interact with many polypeptides, β-barrel outer-membrane proteins are the most prominent substrates. Loss of TF leads to broad outer-membrane defects and premature, cotranslational protein translocation. Whereas in vitro studies suggested that TF is prebound to ribosomes waiting for polypeptides to emerge from the exit channel, we find that in vivo TF engages ribosomes only after ~100 amino acids are translated. Moreover, excess TF interferes with cotranslational removal of the N-terminal formyl methionine. Our studies support a triaging model in which proper protein biogenesis relies on the fine-tuned, sequential engagement of processing, targeting, and folding factors.
Copyright © 2011 Elsevier Inc. All rights reserved.
Figures
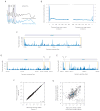
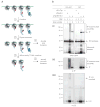
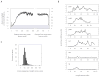
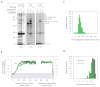
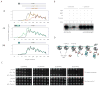
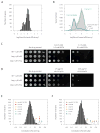
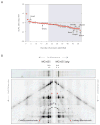
Similar articles
-
Interplay of signal recognition particle and trigger factor at L23 near the nascent chain exit site on the Escherichia coli ribosome.J Cell Biol. 2003 May 26;161(4):679-84. doi: 10.1083/jcb.200302130. Epub 2003 May 19. J Cell Biol. 2003. PMID: 12756233 Free PMC article.
-
Global profiling of SRP interaction with nascent polypeptides.Nature. 2016 Aug 11;536(7615):219-23. doi: 10.1038/nature19070. Epub 2016 Aug 3. Nature. 2016. PMID: 27487212
-
Versatility of trigger factor interactions with ribosome-nascent chain complexes.J Biol Chem. 2010 Sep 3;285(36):27911-23. doi: 10.1074/jbc.M110.134163. Epub 2010 Jul 1. J Biol Chem. 2010. PMID: 20595383 Free PMC article.
-
Ribosome-tethered molecular chaperones: the first line of defense against protein misfolding?Curr Opin Microbiol. 2003 Apr;6(2):157-62. doi: 10.1016/s1369-5274(03)00030-4. Curr Opin Microbiol. 2003. PMID: 12732306 Review.
-
Structure and function of the molecular chaperone Trigger Factor.Biochim Biophys Acta. 2010 Jun;1803(6):650-61. doi: 10.1016/j.bbamcr.2010.01.017. Epub 2010 Feb 2. Biochim Biophys Acta. 2010. PMID: 20132842 Review.
Cited by
-
INRI-seq enables global cell-free analysis of translation initiation and off-target effects of antisense inhibitors.Nucleic Acids Res. 2022 Dec 9;50(22):e128. doi: 10.1093/nar/gkac838. Nucleic Acids Res. 2022. PMID: 36229039 Free PMC article.
-
Computing the functional proteome: recent progress and future prospects for genome-scale models.Curr Opin Biotechnol. 2015 Aug;34:125-34. doi: 10.1016/j.copbio.2014.12.017. Epub 2015 Jan 8. Curr Opin Biotechnol. 2015. PMID: 25576845 Free PMC article. Review.
-
Ligation-free ribosome profiling of cell type-specific translation in the brain.Genome Biol. 2016 Jul 5;17(1):149. doi: 10.1186/s13059-016-1005-1. Genome Biol. 2016. PMID: 27380875 Free PMC article.
-
Hsp33 controls elongation factor-Tu stability and allows Escherichia coli growth in the absence of the major DnaK and trigger factor chaperones.J Biol Chem. 2012 Dec 28;287(53):44435-46. doi: 10.1074/jbc.M112.418525. Epub 2012 Nov 12. J Biol Chem. 2012. PMID: 23148222 Free PMC article.
-
α-Proteobacterial RNA Degradosomes Assemble Liquid-Liquid Phase-Separated RNP Bodies.Mol Cell. 2018 Sep 20;71(6):1027-1039.e14. doi: 10.1016/j.molcel.2018.08.003. Epub 2018 Sep 6. Mol Cell. 2018. PMID: 30197298 Free PMC article.
References
-
- Agashe VR, Guha S, Chang HC, Genevaux P, Hayer-Hartl M, Stemp M, Georgopoulos C, Hartl FU, Barral JM. Function of trigger factor and DnaK in multidomain protein folding: increase in yield at the expense of folding speed. Cell. 2004;117:199–209. - PubMed
-
- Albanèse V, Yam AYW, Baughman J, Parnot C, Frydman J. Systems analyses reveal two chaperone networks with distinct functions in eukaryotic cells. Cell. 2006;124:75–88. - PubMed
-
- Ball LA, Kaesberg P. Cleavage of the N-terminal formylmethionine residue from a bacteriophage coat protein in vitro. J Mol Biol. 1973;79:531–537. - PubMed
-
- Ban N, Nissen P, Hansen J, Moore PB, Steitz TA. The complete atomic structure of the large ribosomal subunit at 2.4 A resolution. Science. 2000;289:905–920. - PubMed
Publication types
MeSH terms
Substances
Associated data
- Actions
- Actions
- Actions
Grants and funding
LinkOut - more resources
Full Text Sources
Other Literature Sources
Molecular Biology Databases
Research Materials
Miscellaneous