Wolbachia induces reactive oxygen species (ROS)-dependent activation of the Toll pathway to control dengue virus in the mosquito Aedes aegypti
- PMID: 22123956
- PMCID: PMC3252928
- DOI: 10.1073/pnas.1116932108
Wolbachia induces reactive oxygen species (ROS)-dependent activation of the Toll pathway to control dengue virus in the mosquito Aedes aegypti
Abstract
Wolbachia are maternally transmitted symbiotic bacteria that can spread within insect populations because of their unique ability to manipulate host reproduction. When introduced to nonnative mosquito hosts, Wolbachia induce resistance to a number of human pathogens, including dengue virus (DENV), Plasmodium, and filarial nematodes, but the molecular mechanism involved is unclear. In this study, we have deciphered how Wolbachia infection affects the Aedes aegypti host in inducing resistance to DENV. The microarray assay indicates that transcripts of genes with functions related to immunity and reduction-oxidation (redox) reactions are up-regulated in Ae. aegypti infected with Wolbachia. Infection with this bacterium leads to induction of oxidative stress and an increased level of reactive oxygen species in its mosquito host. Reactive oxygen species elevation is linked to the activation of the Toll pathway, which is essential in mediating the expression of antioxidants to counterbalance oxidative stress. This immune pathway also is responsible for activation of antimicrobial peptides-defensins and cecropins. We provide evidence that these antimicrobial peptides are involved in inhibition of DENV proliferation in Wolbachia-infected mosquitoes. Utilization of transgenic Ae. aegypti and the RNAi depletion approach has been instrumental in proving the role of defensins and cecropins in the resistance of Wolbachia-infected Ae. aegypti to DENV. These results indicate that a symbiotic bacterium can manipulate the host defense system to facilitate its own persistent infection, resulting in a compromise of the mosquito's ability to host human pathogens. Our discoveries will aid in the development of control strategies for mosquito-transmitted diseases.
Conflict of interest statement
The authors declare no conflict of interest.
Figures
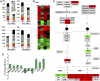
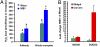
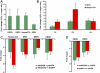
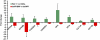
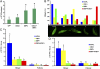
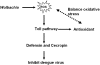
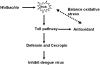
Similar articles
-
Regulation of arginine methyltransferase 3 by a Wolbachia-induced microRNA in Aedes aegypti and its effect on Wolbachia and dengue virus replication.Insect Biochem Mol Biol. 2014 Oct;53:81-8. doi: 10.1016/j.ibmb.2014.08.003. Epub 2014 Aug 23. Insect Biochem Mol Biol. 2014. PMID: 25158106
-
Wolbachia-Based Dengue Virus Inhibition Is Not Tissue-Specific in Aedes aegypti.PLoS Negl Trop Dis. 2016 Nov 17;10(11):e0005145. doi: 10.1371/journal.pntd.0005145. eCollection 2016 Nov. PLoS Negl Trop Dis. 2016. PMID: 27855218 Free PMC article.
-
Upregulation of Aedes aegypti Vago1 by Wolbachia and its effect on dengue virus replication.Insect Biochem Mol Biol. 2018 Jan;92:45-52. doi: 10.1016/j.ibmb.2017.11.008. Epub 2017 Nov 21. Insect Biochem Mol Biol. 2018. PMID: 29157676
-
A comprehensive review of Wolbachia-mediated mechanisms to control dengue virus transmission in Aedes aegypti through innate immune pathways.Front Immunol. 2024 Aug 8;15:1434003. doi: 10.3389/fimmu.2024.1434003. eCollection 2024. Front Immunol. 2024. PMID: 39176079 Free PMC article. Review.
-
Wolbachia-mediated virus blocking in the mosquito vector Aedes aegypti.Curr Opin Insect Sci. 2017 Aug;22:37-44. doi: 10.1016/j.cois.2017.05.005. Epub 2017 May 10. Curr Opin Insect Sci. 2017. PMID: 28805637 Review.
Cited by
-
Dietary cholesterol modulates pathogen blocking by Wolbachia.PLoS Pathog. 2013;9(6):e1003459. doi: 10.1371/journal.ppat.1003459. Epub 2013 Jun 27. PLoS Pathog. 2013. PMID: 23825950 Free PMC article.
-
Burkholderia from Fungus Gardens of Fungus-Growing Ants Produces Antifungals That Inhibit the Specialized Parasite Escovopsis.Appl Environ Microbiol. 2021 Jun 25;87(14):e0017821. doi: 10.1128/AEM.00178-21. Epub 2021 Jun 25. Appl Environ Microbiol. 2021. PMID: 33962985 Free PMC article.
-
Wolbachia Inhibits Binding of Dengue and Zika Viruses to Mosquito Cells.Front Microbiol. 2020 Aug 4;11:1750. doi: 10.3389/fmicb.2020.01750. eCollection 2020. Front Microbiol. 2020. PMID: 32849379 Free PMC article.
-
Diversity and function of bacterial microbiota in the mosquito holobiont.Parasit Vectors. 2013 May 20;6:146. doi: 10.1186/1756-3305-6-146. Parasit Vectors. 2013. PMID: 23688194 Free PMC article. Review.
-
RNAi screening identifies a new Toll from shrimp Litopenaeus vannamei that restricts WSSV infection through activating Dorsal to induce antimicrobial peptides.PLoS Pathog. 2018 Sep 26;14(9):e1007109. doi: 10.1371/journal.ppat.1007109. eCollection 2018 Sep. PLoS Pathog. 2018. PMID: 30256850 Free PMC article.
References
-
- Hill CA, Kafatos FC, Stansfield SK, Collins FH. Arthropod-borne diseases: Vector control in the genomics era. Nat Rev Microbiol. 2005;3:262–268. - PubMed
-
- Halstead SB. Dengue. Lancet. 2007;370:1644–1652. - PubMed
-
- Kyle JL, Harris E. Global spread and persistence of dengue. Annu Rev Microbiol. 2008;62:71–92. - PubMed
-
- Serbus LR, Casper-Lindley C, Landmann F, Sullivan W. The genetics and cell biology of Wolbachia-host interactions. Annu Rev Genet. 2008;42:683–707. - PubMed
Publication types
MeSH terms
Substances
Grants and funding
LinkOut - more resources
Full Text Sources