The giant danio (D. aequipinnatus) as a model of cardiac remodeling and regeneration
- PMID: 22095914
- PMCID: PMC3772730
- DOI: 10.1002/ar.21492
The giant danio (D. aequipinnatus) as a model of cardiac remodeling and regeneration
Abstract
The paucity of mammalian adult cardiac myocytes (CM) proliferation following myocardial infarction (MI) and the remodeling of the necrotic tissue that ensues, result in non-regenerative repair. In contrast, zebrafish (ZF) can regenerate after an apical resection or cryoinjury of the heart. There is considerable interest in models where regeneration proceeds in the presence of necrotic tissue. We have developed and characterized a cautery injury model in the giant danio (GD), a species closely related to ZF, where necrotic tissue remains part of the ventricle, yet regeneration occurs. By light and transmission electron microscopy (TEM), we have documented four temporally overlapping processes: (1) a robust inflammatory response analogous to that observed in MI, (2) concomitant proliferation of epicardial cells leading to wound closure, (3) resorption of necrotic tissue and its replacement by granulation tissue, and (4) regeneration of the myocardial tissue driven by 5-EDU and [(3) H]thymidine incorporating CMs. In conclusion, our data suggest that the GD possesses robust repair mechanisms in the ventricle and can serve as an important model of cardiac inflammation, remodeling and regeneration.
Copyright © 2011 Wiley-Liss, Inc.
Figures
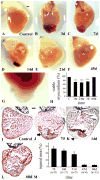
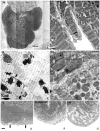
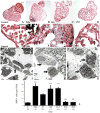
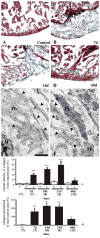
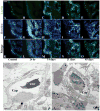
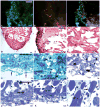
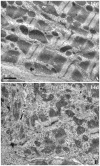
Similar articles
-
Cardiac repair and regenerative potential in the goldfish (Carassius auratus) heart.Comp Biochem Physiol C Toxicol Pharmacol. 2014 Jun;163:14-23. doi: 10.1016/j.cbpc.2014.02.002. Epub 2014 Feb 15. Comp Biochem Physiol C Toxicol Pharmacol. 2014. PMID: 24548889 Free PMC article.
-
Extensive scar formation and regression during heart regeneration after cryoinjury in zebrafish.Development. 2011 May;138(9):1663-74. doi: 10.1242/dev.060897. Epub 2011 Mar 23. Development. 2011. PMID: 21429987
-
Cauterization as a Simple Method for Regeneration Studies in the Zebrafish Heart.J Cardiovasc Dev Dis. 2020 Oct 3;7(4):41. doi: 10.3390/jcdd7040041. J Cardiovasc Dev Dis. 2020. PMID: 33022937 Free PMC article.
-
Cardiac regeneration: epicardial mediated repair.Proc Biol Sci. 2015 Dec 22;282(1821):20152147. doi: 10.1098/rspb.2015.2147. Proc Biol Sci. 2015. PMID: 26702046 Free PMC article. Review.
-
Cell-based therapies for the treatment of myocardial infarction: lessons from cardiac regeneration and repair mechanisms in non-human vertebrates.Heart Fail Rev. 2019 Jan;24(1):133-142. doi: 10.1007/s10741-018-9750-8. Heart Fail Rev. 2019. PMID: 30421074 Review.
Cited by
-
The Macrophage in Cardiac Homeostasis and Disease: JACC Macrophage in CVD Series (Part 4).J Am Coll Cardiol. 2018 Oct 30;72(18):2213-2230. doi: 10.1016/j.jacc.2018.08.2149. J Am Coll Cardiol. 2018. PMID: 30360829 Free PMC article. Review.
-
Can heart function lost to disease be regenerated by therapeutic targeting of cardiac scar tissue?Semin Cell Dev Biol. 2016 Oct;58:41-54. doi: 10.1016/j.semcdb.2016.05.020. Epub 2016 May 24. Semin Cell Dev Biol. 2016. PMID: 27234380 Free PMC article. Review.
-
Reactive oxygen species during heart regeneration in zebrafish: Lessons for future clinical therapies.Wound Repair Regen. 2021 Mar;29(2):211-224. doi: 10.1111/wrr.12892. Epub 2021 Jan 20. Wound Repair Regen. 2021. PMID: 33471940 Free PMC article. Review.
-
Cardiac regenerative capacity: an evolutionary afterthought?Cell Mol Life Sci. 2021 Jun;78(12):5107-5122. doi: 10.1007/s00018-021-03831-9. Epub 2021 May 5. Cell Mol Life Sci. 2021. PMID: 33950316 Free PMC article. Review.
-
Cardiac repair and regenerative potential in the goldfish (Carassius auratus) heart.Comp Biochem Physiol C Toxicol Pharmacol. 2014 Jun;163:14-23. doi: 10.1016/j.cbpc.2014.02.002. Epub 2014 Feb 15. Comp Biochem Physiol C Toxicol Pharmacol. 2014. PMID: 24548889 Free PMC article.
References
-
- Abdullah I, Lepore JJ, et al. MRL mice fail to heal the heart in response to ischemia-reperfusion injury. Wound Repair Regen. 2005;13(2):205–208. - PubMed
-
- Adams DS, Kiyokawa M, Getman ME, Shashoua VE. Genes encoding giant danioand golden shiner ependymin. Neurochem Res. 1996;21:377–384. - PubMed
-
- Bader D, Oberpriller J. Autoradiographic and electron microscopic studies of minced cardiac muscle regeneration in the adult newt, notophthalmus viridescens. JExp Zool. 1979;208:177–193. - PubMed
Publication types
MeSH terms
Substances
Grants and funding
LinkOut - more resources
Full Text Sources