Angiotensin II increases activity of the epithelial Na+ channel (ENaC) in distal nephron additively to aldosterone
- PMID: 22086923
- PMCID: PMC3249120
- DOI: 10.1074/jbc.M111.298919
Angiotensin II increases activity of the epithelial Na+ channel (ENaC) in distal nephron additively to aldosterone
Abstract
Dietary salt intake controls epithelial Na+ channel (ENaC)-mediated Na+ reabsorption in the distal nephron by affecting status of the renin-angiotensin-aldosterone system (RAAS). Whereas regulation of ENaC by aldosterone is generally accepted, little is known about whether other components of RAAS, such as angiotensin II (Ang II), have nonredundant to aldosterone-stimulatory actions on ENaC. We combined patch clamp electrophysiology and immunohistochemistry in freshly isolated split-opened distal nephrons of mice to determine the mechanism and molecular signaling pathway of Ang II regulation of ENaC. We found that Ang II acutely increases ENaC Po, whereas prolonged exposure to Ang II also induces translocation of α-ENaC toward the apical membrane in situ. Ang II actions on ENaC Po persist in the presence of saturated mineralocorticoid status. Moreover, aldosterone fails to stimulate ENaC acutely, suggesting that Ang II and aldosterone have different time frames of ENaC activation. AT1 but not AT2 receptors mediate Ang II actions on ENaC. Unlike its effect in vasculature, Ang II did not increase [Ca2+]i in split-opened distal nephrons as demonstrated using ratiometric Fura-2-based microscopy. However, application of Ang II to mpkCCDc14 cells resulted in generation of reactive oxygen species, as probed with fluorescent methods. Consistently, inhibiting NADPH oxidase with apocynin abolished Ang II-mediated increases in ENaC Po in murine distal nephron. Therefore, we concluded that Ang II directly regulates ENaC activity in the distal nephron, and this effect complements regulation of ENaC by aldosterone. We propose that stimulation of AT1 receptors with subsequent activation of NADPH oxidase signaling pathway mediates Ang II actions on ENaC.
Figures
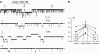
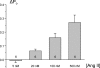
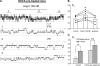
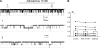
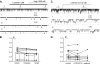
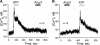
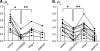
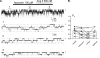
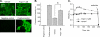
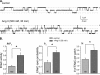
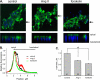
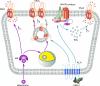
Similar articles
-
NOXA1-dependent NADPH oxidase 1 signaling mediates angiotensin II activation of the epithelial sodium channel.Am J Physiol Renal Physiol. 2022 Dec 1;323(6):F633-F641. doi: 10.1152/ajprenal.00107.2022. Epub 2022 Oct 6. Am J Physiol Renal Physiol. 2022. PMID: 36201326 Free PMC article.
-
Chronic angiotensin II infusion drives extensive aldosterone-independent epithelial Na+ channel activation.Hypertension. 2013 Dec;62(6):1111-1122. doi: 10.1161/HYPERTENSIONAHA.113.01797. Epub 2013 Sep 23. Hypertension. 2013. PMID: 24060890 Free PMC article.
-
Bradykinin acutely inhibits activity of the epithelial Na+ channel in mammalian aldosterone-sensitive distal nephron.Am J Physiol Renal Physiol. 2011 May;300(5):F1105-15. doi: 10.1152/ajprenal.00606.2010. Epub 2011 Feb 16. Am J Physiol Renal Physiol. 2011. PMID: 21325499 Free PMC article.
-
Direct activation of ENaC by angiotensin II: recent advances and new insights.Curr Hypertens Rep. 2013 Feb;15(1):17-24. doi: 10.1007/s11906-012-0316-1. Curr Hypertens Rep. 2013. PMID: 23180052 Free PMC article. Review.
-
Direct regulation of ENaC by bradykinin in the distal nephron. Implications for renal sodium handling.Curr Opin Nephrol Hypertens. 2014 Mar;23(2):122-9. doi: 10.1097/01.mnh.0000441053.81339.61. Curr Opin Nephrol Hypertens. 2014. PMID: 24378775 Free PMC article. Review.
Cited by
-
Oxidative stress in hypertension: role of the kidney.Antioxid Redox Signal. 2014 Jan 1;20(1):74-101. doi: 10.1089/ars.2013.5259. Epub 2013 Apr 30. Antioxid Redox Signal. 2014. PMID: 23472618 Free PMC article. Review.
-
Salt-dependent inhibition of epithelial Na+ channel-mediated sodium reabsorption in the aldosterone-sensitive distal nephron by bradykinin.Hypertension. 2012 Nov;60(5):1234-41. doi: 10.1161/HYPERTENSIONAHA.112.200469. Epub 2012 Oct 1. Hypertension. 2012. PMID: 23033373 Free PMC article.
-
The role of transforming growth factor β1 in the regulation of blood pressure.Curr Hypertens Rev. 2014;10(4):223-38. doi: 10.2174/157340211004150319123313. Curr Hypertens Rev. 2014. PMID: 25801626 Free PMC article. Review.
-
Podocyte Injury Augments Intrarenal Angiotensin II Generation and Sodium Retention in a Megalin-Dependent Manner.Hypertension. 2019 Sep;74(3):509-517. doi: 10.1161/HYPERTENSIONAHA.118.12352. Epub 2019 Jul 29. Hypertension. 2019. PMID: 31352823 Free PMC article.
-
The renin-angiotensin-aldosterone system: a crossroad from arterial hypertension to heart failure.Heart Fail Rev. 2020 Jan;25(1):31-42. doi: 10.1007/s10741-019-09855-5. Heart Fail Rev. 2020. PMID: 31512149 Review.
References
Publication types
MeSH terms
Substances
Grants and funding
LinkOut - more resources
Full Text Sources
Other Literature Sources
Research Materials
Miscellaneous