Adhesive F-actin waves: a novel integrin-mediated adhesion complex coupled to ventral actin polymerization
- PMID: 22069459
- PMCID: PMC3206032
- DOI: 10.1371/journal.pone.0026631
Adhesive F-actin waves: a novel integrin-mediated adhesion complex coupled to ventral actin polymerization
Abstract
At the leading lamellipodium of migrating cells, protrusion of an Arp2/3-nucleated actin network is coupled to formation of integrin-based adhesions, suggesting that Arp2/3-mediated actin polymerization and integrin-dependent adhesion may be mechanistically linked. Arp2/3 also mediates actin polymerization in structures distinct from the lamellipodium, in "ventral F-actin waves" that propagate as spots and wavefronts along the ventral plasma membrane. Here we show that integrins engage the extracellular matrix downstream of ventral F-actin waves in several mammalian cell lines as well as in primary mouse embryonic fibroblasts. These "adhesive F-actin waves" require a cycle of integrin engagement and disengagement to the extracellular matrix for their formation and propagation, and exhibit morphometry and a hierarchical assembly and disassembly mechanism distinct from other integrin-containing structures. After Arp2/3-mediated actin polymerization, zyxin and VASP are co-recruited to adhesive F-actin waves, followed by paxillin and vinculin, and finally talin and integrin. Adhesive F-actin waves thus represent a previously uncharacterized integrin-based adhesion complex associated with Arp2/3-mediated actin polymerization.
Conflict of interest statement
Figures
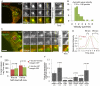
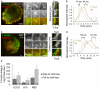
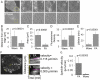
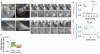
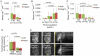
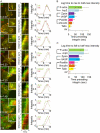
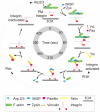
Similar articles
-
Talin-activated vinculin interacts with branched actin networks to initiate bundles.Elife. 2020 Nov 13;9:e53990. doi: 10.7554/eLife.53990. Elife. 2020. PMID: 33185186 Free PMC article.
-
Dynamics and distribution of paxillin, vinculin, zyxin and VASP depend on focal adhesion location and orientation.Sci Rep. 2019 Jul 18;9(1):10460. doi: 10.1038/s41598-019-46905-2. Sci Rep. 2019. PMID: 31320676 Free PMC article.
-
Recruitment of the Arp2/3 complex to vinculin: coupling membrane protrusion to matrix adhesion.J Cell Biol. 2002 Dec 9;159(5):881-91. doi: 10.1083/jcb.200206043. Epub 2002 Dec 9. J Cell Biol. 2002. PMID: 12473693 Free PMC article.
-
Integrin-mediated cell adhesion: the cytoskeletal connection.Biochem Soc Symp. 1999;65:79-99. Biochem Soc Symp. 1999. PMID: 10320934 Review.
-
The Actin Network Interfacing Diverse Integrin-Mediated Adhesions.Biomolecules. 2023 Feb 4;13(2):294. doi: 10.3390/biom13020294. Biomolecules. 2023. PMID: 36830665 Free PMC article. Review.
Cited by
-
Differential cellular responses to adhesive interactions with galectin-8- and fibronectin-coated substrates.J Cell Sci. 2021 Apr 15;134(8):jcs252221. doi: 10.1242/jcs.252221. Epub 2021 Apr 27. J Cell Sci. 2021. PMID: 33722978 Free PMC article.
-
ARF6 promotes the formation of Rac1 and WAVE-dependent ventral F-actin rosettes in breast cancer cells in response to epidermal growth factor.PLoS One. 2015 Mar 23;10(3):e0121747. doi: 10.1371/journal.pone.0121747. eCollection 2015. PLoS One. 2015. PMID: 25799492 Free PMC article.
-
Abundant Focal Adhesion Kinase Causes Aberrant Neuronal Migration Via Its Phosphorylation at Tyr925.J Mol Neurosci. 2018 Jan;64(1):102-110. doi: 10.1007/s12031-017-1010-1. Epub 2017 Dec 5. J Mol Neurosci. 2018. PMID: 29209901
-
Moving towards a paradigm: common mechanisms of chemotactic signaling in Dictyostelium and mammalian leukocytes.Cell Mol Life Sci. 2014 Oct;71(19):3711-47. doi: 10.1007/s00018-014-1638-8. Epub 2014 May 21. Cell Mol Life Sci. 2014. PMID: 24846395 Free PMC article. Review.
-
Excitable Signal Transduction Networks in Directed Cell Migration.Annu Rev Cell Dev Biol. 2017 Oct 6;33:103-125. doi: 10.1146/annurev-cellbio-100616-060739. Epub 2017 Aug 9. Annu Rev Cell Dev Biol. 2017. PMID: 28793794 Free PMC article. Review.
References
-
- Serrels B, Serrels A, Brunton VG, Holt M, McLean GW, et al. Focal adhesion kinase controls actin assembly via a FERM-mediated interaction with the Arp2/3 complex. Nat Cell Biol. 2007;9:1046–1056. - PubMed
-
- Goley ED, Welch MD. The ARP2/3 complex: an actin nucleator comes of age. Nat Rev Mol Cell Biol. 2006;7:713–726. - PubMed
Publication types
MeSH terms
Substances
LinkOut - more resources
Full Text Sources
Other Literature Sources