The vinculin C-terminal hairpin mediates F-actin bundle formation, focal adhesion, and cell mechanical properties
- PMID: 22052910
- PMCID: PMC3247952
- DOI: 10.1074/jbc.M111.244293
The vinculin C-terminal hairpin mediates F-actin bundle formation, focal adhesion, and cell mechanical properties
Abstract
Vinculin is an essential and highly conserved cell adhesion protein, found at both focal adhesions and adherens junctions, where it couples integrins or cadherins to the actin cytoskeleton. Vinculin is involved in controlling cell shape, motility, and cell survival, and has more recently been shown to play a role in force transduction. The tail domain of vinculin (Vt) contains determinants necessary for binding and bundling of actin filaments. Actin binding to Vt has been proposed to induce formation of a Vt dimer that is necessary for cross-linking actin filaments. Results from this study provide additional support for actin-induced Vt self-association. Moreover, the actin-induced Vt dimer appears distinct from the dimer formed in the absence of actin. To better characterize the role of the Vt strap and carboxyl terminus (CT) in actin binding, Vt self-association, and actin bundling, we employed smaller amino-terminal (NT) and CT deletions that do not perturb the structural integrity of Vt. Although both NT and CT deletions retain actin binding, removal of the CT hairpin (1061-1066) selectively impairs actin bundling in vitro. Moreover, expression of vinculin lacking the CT hairpin in vinculin knock-out murine embryonic fibroblasts affects the number of focal adhesions formed, cell spreading as well as cellular stiffening in response to mechanical force.
Figures
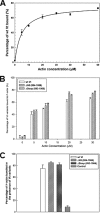
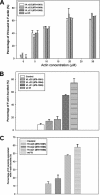
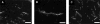
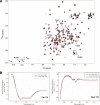
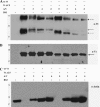
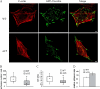
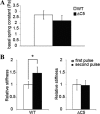
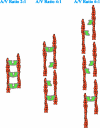
Comment in
-
Vinculin regulation of F-actin bundle formation: what does it mean for the cell?Cell Adh Migr. 2013 Mar-Apr;7(2):219-25. doi: 10.4161/cam.23184. Epub 2013 Jan 10. Cell Adh Migr. 2013. PMID: 23307141 Free PMC article.
Similar articles
-
Vinculin regulation of F-actin bundle formation: what does it mean for the cell?Cell Adh Migr. 2013 Mar-Apr;7(2):219-25. doi: 10.4161/cam.23184. Epub 2013 Jan 10. Cell Adh Migr. 2013. PMID: 23307141 Free PMC article.
-
Lipid binding to the tail domain of vinculin: specificity and the role of the N and C termini.J Biol Chem. 2009 Mar 13;284(11):7223-31. doi: 10.1074/jbc.M807842200. Epub 2008 Dec 24. J Biol Chem. 2009. PMID: 19110481 Free PMC article.
-
Identification of an actin binding surface on vinculin that mediates mechanical cell and focal adhesion properties.Structure. 2014 May 6;22(5):697-706. doi: 10.1016/j.str.2014.03.002. Epub 2014 Mar 27. Structure. 2014. PMID: 24685146 Free PMC article.
-
New insights into vinculin function and regulation.Int Rev Cell Mol Biol. 2011;287:191-231. doi: 10.1016/B978-0-12-386043-9.00005-0. Int Rev Cell Mol Biol. 2011. PMID: 21414589 Free PMC article. Review.
-
Vinculin and metavinculin: oligomerization and interactions with F-actin.FEBS Lett. 2013 Apr 17;587(8):1220-9. doi: 10.1016/j.febslet.2013.02.042. Epub 2013 Mar 1. FEBS Lett. 2013. PMID: 23466368 Review.
Cited by
-
The C-terminal tail domain of metavinculin, vinculin's splice variant, severs actin filaments.J Cell Biol. 2012 May 28;197(5):585-93. doi: 10.1083/jcb.201111046. Epub 2012 May 21. J Cell Biol. 2012. PMID: 22613835 Free PMC article.
-
Vinculin and metavinculin exhibit distinct effects on focal adhesion properties, cell migration, and mechanotransduction.PLoS One. 2019 Sep 4;14(9):e0221962. doi: 10.1371/journal.pone.0221962. eCollection 2019. PLoS One. 2019. PMID: 31483833 Free PMC article.
-
Vinculin regulation of F-actin bundle formation: what does it mean for the cell?Cell Adh Migr. 2013 Mar-Apr;7(2):219-25. doi: 10.4161/cam.23184. Epub 2013 Jan 10. Cell Adh Migr. 2013. PMID: 23307141 Free PMC article.
-
Complete Model of Vinculin Suggests the Mechanism of Activation by Helical Super-Bundle Unfurling.Protein J. 2022 Feb;41(1):55-70. doi: 10.1007/s10930-022-10040-1. Epub 2022 Jan 10. Protein J. 2022. PMID: 35006498
-
Cardiomyopathy Mutations in Metavinculin Disrupt Regulation of Vinculin-Induced F-Actin Assemblies.J Mol Biol. 2019 Apr 5;431(8):1604-1618. doi: 10.1016/j.jmb.2019.02.024. Epub 2019 Mar 5. J Mol Biol. 2019. PMID: 30844403 Free PMC article.
References
-
- Janmey P. A., McCulloch C. A. (2007) Annu. Rev. Biomed. Eng. 9, 1–34 - PubMed
-
- Geiger B., Spatz J. P., Bershadsky A. D. (2009) Nat. Rev. Mol. Cell Biol. 10, 21–33 - PubMed
-
- Geiger B., Bershadsky A. (2001) Curr. Opin. Cell Biol. 13, 584–592 - PubMed
-
- Ziegler W. H., Liddington R. C., Critchley D. R. (2006) Trends Cell Biol. 16, 453–460 - PubMed
Publication types
MeSH terms
Substances
Grants and funding
- R01 GM081764/GM/NIGMS NIH HHS/United States
- R01 GM080568-04/GM/NIGMS NIH HHS/United States
- R01 GM081764-01A2/GM/NIGMS NIH HHS/United States
- R01 GM080568/GM/NIGMS NIH HHS/United States
- R01 GM029860/GM/NIGMS NIH HHS/United States
- R01 GM080568-02/GM/NIGMS NIH HHS/United States
- GM081764/GM/NIGMS NIH HHS/United States
- GM080568/GM/NIGMS NIH HHS/United States
- R01 GM081764-02/GM/NIGMS NIH HHS/United States
- R01 GM080568-03/GM/NIGMS NIH HHS/United States
- GM029860/GM/NIGMS NIH HHS/United States
- R01 GM080568-01A1/GM/NIGMS NIH HHS/United States
LinkOut - more resources
Full Text Sources
Research Materials