Activation mechanism of the β2-adrenergic receptor
- PMID: 22031696
- PMCID: PMC3219117
- DOI: 10.1073/pnas.1110499108
Activation mechanism of the β2-adrenergic receptor
Abstract
A third of marketed drugs act by binding to a G-protein-coupled receptor (GPCR) and either triggering or preventing receptor activation. Although recent crystal structures have provided snapshots of both active and inactive functional states of GPCRs, these structures do not reveal the mechanism by which GPCRs transition between these states. Here we propose an activation mechanism for the β(2)-adrenergic receptor, a prototypical GPCR, based on atomic-level simulations in which an agonist-bound receptor transitions spontaneously from the active to the inactive crystallographically observed conformation. A loosely coupled allosteric network, comprising three regions that can each switch individually between multiple distinct conformations, links small perturbations at the extracellular drug-binding site to large conformational changes at the intracellular G-protein-binding site. Our simulations also exhibit an intermediate that may represent a receptor conformation to which a G protein binds during activation, and suggest that the first structural changes during receptor activation often take place on the intracellular side of the receptor, far from the drug-binding site. By capturing this fundamental signaling process in atomic detail, our results may provide a foundation for the design of drugs that control receptor signaling more precisely by stabilizing specific receptor conformations.
Conflict of interest statement
The authors declare no conflict of interest.
Figures
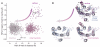
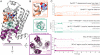
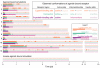
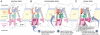
Similar articles
-
Simulations of biased agonists in the β(2) adrenergic receptor with accelerated molecular dynamics.Biochemistry. 2013 Aug 20;52(33):5593-603. doi: 10.1021/bi400499n. Epub 2013 Aug 7. Biochemistry. 2013. PMID: 23879802 Free PMC article.
-
Allosteric nanobodies reveal the dynamic range and diverse mechanisms of G-protein-coupled receptor activation.Nature. 2016 Jul 21;535(7612):448-52. doi: 10.1038/nature18636. Epub 2016 Jul 13. Nature. 2016. PMID: 27409812 Free PMC article.
-
Molecular dynamics simulations of the effect of the G-protein and diffusible ligands on the β2-adrenergic receptor.J Mol Biol. 2011 Dec 9;414(4):611-23. doi: 10.1016/j.jmb.2011.10.015. Epub 2011 Oct 20. J Mol Biol. 2011. PMID: 22037586
-
Structural features of β2 adrenergic receptor: crystal structures and beyond.Mol Cells. 2015;38(2):105-11. doi: 10.14348/molcells.2015.2301. Epub 2014 Dec 24. Mol Cells. 2015. PMID: 25537861 Free PMC article. Review.
-
The role of protein dynamics in GPCR function: insights from the β2AR and rhodopsin.Curr Opin Cell Biol. 2014 Apr;27:136-43. doi: 10.1016/j.ceb.2014.01.008. Epub 2014 Feb 17. Curr Opin Cell Biol. 2014. PMID: 24534489 Free PMC article. Review.
Cited by
-
Molecular Dynamics (MD) Simulations Provide Insights into the Activation Mechanisms of 5-HT2A Receptors.Molecules. 2024 Oct 18;29(20):4935. doi: 10.3390/molecules29204935. Molecules. 2024. PMID: 39459303 Free PMC article.
-
Deciphering collaborative sidechain motions in proteins during molecular dynamics simulations.Sci Rep. 2020 Sep 28;10(1):15901. doi: 10.1038/s41598-020-72766-1. Sci Rep. 2020. PMID: 32985550 Free PMC article.
-
β-adrenergic receptor regulates embryonic epithelial extensibility through actomyosin inhibition.iScience. 2023 Nov 15;26(12):108469. doi: 10.1016/j.isci.2023.108469. eCollection 2023 Dec 15. iScience. 2023. PMID: 38213788 Free PMC article.
-
Revealing the favorable dissociation pathway of type II kinase inhibitors via enhanced sampling simulations and two-end-state calculations.Sci Rep. 2015 Feb 13;5:8457. doi: 10.1038/srep08457. Sci Rep. 2015. PMID: 25678308 Free PMC article.
-
The Third Extracellular Loop of Mammalian Odorant Receptors Is Involved in Ligand Binding.Int J Mol Sci. 2022 Oct 18;23(20):12501. doi: 10.3390/ijms232012501. Int J Mol Sci. 2022. PMID: 36293357 Free PMC article.
References
-
- Rasmussen SGF, et al. Crystal structure of the β2 adrenergic receptor-Gs protein complex. Nature. 2011 10.1038/nature10361. - DOI - PMC - PubMed
-
- Palczewski K, et al. Crystal structure of rhodopsin: A G protein-coupled receptor. Science. 2000;289:739–745. - PubMed
-
- Choe HW, et al. Crystal structure of metarhodopsin II. Nature. 2011;471:651–655. - PubMed
MeSH terms
Substances
LinkOut - more resources
Full Text Sources
Other Literature Sources