Progranulin, a glycoprotein deficient in frontotemporal dementia, is a novel substrate of several protein disulfide isomerase family proteins
- PMID: 22028881
- PMCID: PMC3196579
- DOI: 10.1371/journal.pone.0026454
Progranulin, a glycoprotein deficient in frontotemporal dementia, is a novel substrate of several protein disulfide isomerase family proteins
Abstract
The reduced production or activity of the cysteine-rich glycoprotein progranulin is responsible for about 20% of cases of familial frontotemporal dementia. However, little is known about the molecular mechanisms that govern the level and secretion of progranulin. Here we show that progranulin is expressed in mouse cortical neurons and more prominently in mouse microglia in culture and is abundant in the endoplasmic reticulum (ER) and Golgi. Using chemical crosslinking, immunoprecipitation, and mass spectrometry, we found that progranulin is bound to a network of ER Ca(2+)-binding chaperones including BiP, calreticulin, GRP94, and four members of the protein disulfide isomerase (PDI) family. Loss of ERp57 inhibits progranulin secretion. Thus, progranulin is a novel substrate of several PDI family proteins and modulation of the ER chaperone network may be a therapeutic target for controlling progranulin secretion.
Conflict of interest statement
Figures
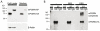
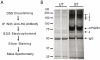
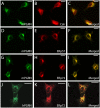
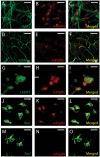
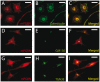
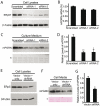
Similar articles
-
Behavioral deficits and progressive neuropathology in progranulin-deficient mice: a mouse model of frontotemporal dementia.FASEB J. 2010 Dec;24(12):4639-47. doi: 10.1096/fj.10-161471. Epub 2010 Jul 28. FASEB J. 2010. PMID: 20667979 Free PMC article.
-
Microglial NFκB-TNFα hyperactivation induces obsessive-compulsive behavior in mouse models of progranulin-deficient frontotemporal dementia.Proc Natl Acad Sci U S A. 2017 May 9;114(19):5029-5034. doi: 10.1073/pnas.1700477114. Epub 2017 Apr 24. Proc Natl Acad Sci U S A. 2017. PMID: 28438992 Free PMC article.
-
Restoring neuronal progranulin reverses deficits in a mouse model of frontotemporal dementia.Brain. 2017 May 1;140(5):1447-1465. doi: 10.1093/brain/awx060. Brain. 2017. PMID: 28379303 Free PMC article.
-
Progranulin: at the interface of neurodegenerative and metabolic diseases.Trends Endocrinol Metab. 2013 Dec;24(12):597-606. doi: 10.1016/j.tem.2013.08.003. Epub 2013 Sep 10. Trends Endocrinol Metab. 2013. PMID: 24035620 Free PMC article. Review.
-
Potential mechanisms of progranulin-deficient FTLD.J Mol Neurosci. 2011 Nov;45(3):574-82. doi: 10.1007/s12031-011-9622-3. Epub 2011 Sep 3. J Mol Neurosci. 2011. PMID: 21892758 Free PMC article. Review.
Cited by
-
Proteomics Approaches for Biomarker and Drug Target Discovery in ALS and FTD.Front Neurosci. 2019 Jun 11;13:548. doi: 10.3389/fnins.2019.00548. eCollection 2019. Front Neurosci. 2019. PMID: 31244593 Free PMC article. Review.
-
Mice Treated Subcutaneously with Mouse LPS-Converted PrPres or LPS Alone Showed Brain Gene Expression Profiles Characteristic of Prion Disease.Vet Sci. 2021 Sep 21;8(9):200. doi: 10.3390/vetsci8090200. Vet Sci. 2021. PMID: 34564594 Free PMC article.
-
Intracellular Proteolysis of Progranulin Generates Stable, Lysosomal Granulins that Are Haploinsufficient in Patients with Frontotemporal Dementia Caused by GRN Mutations.eNeuro. 2017 Aug 18;4(4):ENEURO.0100-17.2017. doi: 10.1523/ENEURO.0100-17.2017. eCollection 2017 Jul-Aug. eNeuro. 2017. PMID: 28828399 Free PMC article.
-
Progranulin: A conductor of receptors orchestra, a chaperone of lysosomal enzymes and a therapeutic target for multiple diseases.Cytokine Growth Factor Rev. 2019 Feb;45:53-64. doi: 10.1016/j.cytogfr.2019.01.002. Epub 2019 Jan 30. Cytokine Growth Factor Rev. 2019. PMID: 30733059 Free PMC article. Review.
-
Multifunctional molecule ERp57: From cancer to neurodegenerative diseases.Pharmacol Ther. 2018 Jan;181:34-48. doi: 10.1016/j.pharmthera.2017.07.011. Epub 2017 Jul 16. Pharmacol Ther. 2018. PMID: 28723413 Free PMC article. Review.
References
-
- Boxer AL, Miller BL. Clinical features of frontotemporal dementia. Alzheimer Dis Assoc Disord. 2005;19(Suppl 1):S36. - PubMed
-
- Baker M, Mackenzie IR, Pickering-Brown SM, Gass J, Rademakers R, et al. Mutations in progranulin cause tau-negative frontotemporal dementia linked to chromosome 17. Nature. 2006;442:916–919. - PubMed
-
- Cruts M, Gijselinck I, van der Zee J, Engelborghs S, Wils H, et al. Null mutations in progranulin cause ubiquitin-positive frontotemporal dementia linked to chromosome 17q21. Nature. 2006;442:920–924. - PubMed
-
- Gijselinck I, van der Zee J, Engelborghs S, Goossens D, Peeters K, et al. Progranulin locus deletion in frontotemporal dementia. Hum Mutat. 2008;29:53–58. - PubMed
Publication types
MeSH terms
Substances
Grants and funding
LinkOut - more resources
Full Text Sources
Other Literature Sources
Molecular Biology Databases
Research Materials
Miscellaneous