Determinants of relapse periodicity in Plasmodium vivax malaria
- PMID: 21989376
- PMCID: PMC3228849
- DOI: 10.1186/1475-2875-10-297
Determinants of relapse periodicity in Plasmodium vivax malaria
Abstract
Plasmodium vivax is a major cause of febrile illness in endemic areas of Asia, Central and South America, and the horn of Africa. Plasmodium vivax infections are characterized by relapses of malaria arising from persistent liver stages of the parasite (hypnozoites) which can be prevented only by 8-aminoquinoline anti-malarials. Tropical P. vivax relapses at three week intervals if rapidly eliminated anti-malarials are given for treatment, whereas in temperate regions and parts of the sub-tropics P. vivax infections are characterized either by a long incubation or a long-latency period between illness and relapse - in both cases approximating 8-10 months. The epidemiology of the different relapse phenotypes has not been defined adequately despite obvious relevance to malaria control and elimination. The number of sporozoites inoculated by the anopheline mosquito is an important determinant of both the timing and the number of relapses. The intervals between relapses display a remarkable periodicity which has not been explained. Evidence is presented that the proportion of patients who have successive relapses is relatively constant and that the factor which activates hypnozoites and leads to regular interval relapse in vivax malaria is the systemic febrile illness itself. It is proposed that in endemic areas a large proportion of the population harbours latent hypnozoites which can be activated by a systemic illness such as vivax or falciparum malaria. This explains the high rates of vivax following falciparum malaria, the high proportion of heterologous genotypes in relapses, the higher rates of relapse in people living in endemic areas compared with artificial infection studies, and, by facilitating recombination between different genotypes, contributes to P. vivax genetic diversity particularly in low transmission settings. Long-latency P. vivax phenotypes may be more widespread and more prevalent than currently thought. These observations have important implications for the assessment of radical treatment efficacy and for malaria control and elimination.
Figures
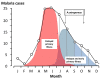
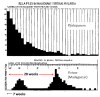
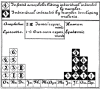
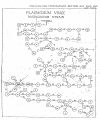
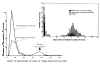
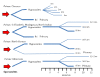
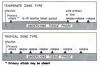
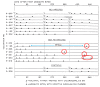
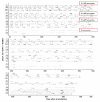
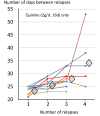
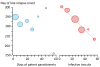
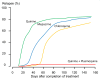
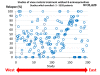
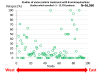
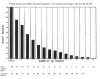
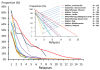
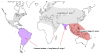
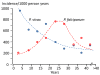
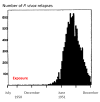
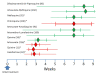
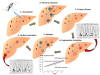
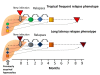
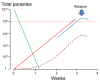
Similar articles
-
Relapse.Adv Parasitol. 2012;80:113-50. doi: 10.1016/B978-0-12-397900-1.00002-5. Adv Parasitol. 2012. PMID: 23199487 Review.
-
Genotyping of Plasmodium vivax reveals both short and long latency relapse patterns in Kolkata.PLoS One. 2012;7(7):e39645. doi: 10.1371/journal.pone.0039645. Epub 2012 Jul 13. PLoS One. 2012. PMID: 22808048 Free PMC article. Clinical Trial.
-
Population-level estimates of the proportion of Plasmodium vivax blood-stage infections attributable to relapses among febrile patients attending Adama Malaria Diagnostic Centre, East Shoa Zone, Oromia, Ethiopia.Malar J. 2017 Jul 27;16(1):301. doi: 10.1186/s12936-017-1944-3. Malar J. 2017. PMID: 28750669 Free PMC article.
-
Plasmodium vivax Latent Liver Stage Infection and Relapse: Biological Insights and New Experimental Tools.Annu Rev Microbiol. 2021 Oct 8;75:87-106. doi: 10.1146/annurev-micro-032421-061155. Epub 2021 Jul 1. Annu Rev Microbiol. 2021. PMID: 34196569 Review.
-
The empirical support for the radical cure strategy for eliminating Plasmodium vivax in China.BMC Med. 2022 Jan 21;20(1):17. doi: 10.1186/s12916-021-02214-y. BMC Med. 2022. PMID: 35057816 Free PMC article.
Cited by
-
Plasmodium vivax merozoite surface protein-3 (PvMSP3): expression of an 11 member multigene family in blood-stage parasites.PLoS One. 2013 May 23;8(5):e63888. doi: 10.1371/journal.pone.0063888. Print 2013. PLoS One. 2013. PMID: 23717506 Free PMC article.
-
Tafenoquine and its potential in the treatment and relapse prevention of Plasmodium vivax malaria: the evidence to date.Drug Des Devel Ther. 2016 Jul 26;10:2387-99. doi: 10.2147/DDDT.S61443. eCollection 2016. Drug Des Devel Ther. 2016. PMID: 27528800 Free PMC article. Review.
-
Analysis of asymptomatic and clinical malaria in urban and suburban settings of southwestern Ethiopia in the context of sustaining malaria control and approaching elimination.Malar J. 2016 Apr 30;15:250. doi: 10.1186/s12936-016-1298-2. Malar J. 2016. PMID: 27129785 Free PMC article.
-
Liver-stage fate determination in Plasmodium vivax parasites: Characterization of schizont growth and hypnozoite fating from patient isolates.Front Microbiol. 2022 Sep 23;13:976606. doi: 10.3389/fmicb.2022.976606. eCollection 2022. Front Microbiol. 2022. PMID: 36212849 Free PMC article.
-
Host kinase regulation of Plasmodium vivax dormant and replicating liver stages.bioRxiv [Preprint]. 2023 Nov 13:2023.11.13.566868. doi: 10.1101/2023.11.13.566868. bioRxiv. 2023. PMID: 38014051 Free PMC article. Preprint.
References
-
- Laveran A. Nouveau parasite du sang. Bull Acad Natl Med. 1980;10:1235–1236.
-
- Golgi C. Sulle febbri malariche estivo-autumnali di Roma. Gazz Med Pavia. 1983;10:481–559.
-
- Grassi B, Feletti R. Parasites malariques chez les oiseaux. Arch Ital Biol. 1890;10:297–300.
Publication types
MeSH terms
Grants and funding
LinkOut - more resources
Full Text Sources
Other Literature Sources