Extended surfaces modulate hydrophobic interactions of neighboring solutes
- PMID: 21987795
- PMCID: PMC3203787
- DOI: 10.1073/pnas.1110703108
Extended surfaces modulate hydrophobic interactions of neighboring solutes
Abstract
Interfaces are a most common motif in complex systems. To understand how the presence of interfaces affects hydrophobic phenomena, we use molecular simulations and theory to study hydration of solutes at interfaces. The solutes range in size from subnanometer to a few nanometers. The interfaces are self-assembled monolayers with a range of chemistries, from hydrophilic to hydrophobic. We show that the driving force for assembly in the vicinity of a hydrophobic surface is weaker than that in bulk water and decreases with increasing temperature, in contrast to that in the bulk. We explain these distinct features in terms of an interplay between interfacial fluctuations and excluded volume effects--the physics encoded in Lum-Chandler-Weeks theory [Lum K, Chandler D, Weeks JD (1999) J Phys Chem B 103:4570-4577]. Our results suggest a catalytic role for hydrophobic interfaces in the unfolding of proteins, for example, in the interior of chaperonins and in amyloid formation.
Conflict of interest statement
The authors declare no conflict of interest.
Figures
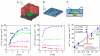
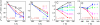
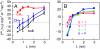
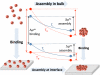
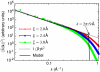


Similar articles
-
Hydrophobic hydration from small to large lengthscales: Understanding and manipulating the crossover.Proc Natl Acad Sci U S A. 2005 Jul 5;102(27):9475-80. doi: 10.1073/pnas.0504089102. Epub 2005 Jun 22. Proc Natl Acad Sci U S A. 2005. PMID: 15972804 Free PMC article.
-
On the thermodynamics and kinetics of hydrophobic interactions at interfaces.J Phys Chem B. 2013 Sep 5;117(35):10261-70. doi: 10.1021/jp4050513. Epub 2013 Aug 22. J Phys Chem B. 2013. PMID: 23906438
-
Enthalpy-entropy contributions to salt and osmolyte effects on molecular-scale hydrophobic hydration and interactions.J Phys Chem B. 2008 May 8;112(18):5661-70. doi: 10.1021/jp073485n. J Phys Chem B. 2008. PMID: 18447346
-
Hydrophobicity of proteins and interfaces: insights from density fluctuations.Annu Rev Chem Biomol Eng. 2011;2:147-71. doi: 10.1146/annurev-chembioeng-061010-114156. Annu Rev Chem Biomol Eng. 2011. PMID: 22432614 Review.
-
Reconciling the understanding of 'hydrophobicity' with physics-based models of proteins.J Phys Condens Matter. 2016 Mar 2;28(8):083003. doi: 10.1088/0953-8984/28/8/083003. Epub 2016 Feb 2. J Phys Condens Matter. 2016. PMID: 26836518 Free PMC article. Review.
Cited by
-
Pre-transition effects mediate forces of assembly between transmembrane proteins.Elife. 2016 Feb 24;5:e13150. doi: 10.7554/eLife.13150. Elife. 2016. PMID: 26910009 Free PMC article.
-
A Roadmap for Building Waterborne Virus Traps.JACS Au. 2022 Oct 4;2(10):2205-2221. doi: 10.1021/jacsau.2c00377. eCollection 2022 Oct 24. JACS Au. 2022. PMID: 36311831 Free PMC article. Review.
-
Necessity of capillary modes in a minimal model of nanoscale hydrophobic solvation.Proc Natl Acad Sci U S A. 2016 Apr 19;113(16):E2224-30. doi: 10.1073/pnas.1513659113. Epub 2016 Mar 8. Proc Natl Acad Sci U S A. 2016. PMID: 26957607 Free PMC article.
-
Water-mediated ion-ion interactions are enhanced at the water vapor-liquid interface.Proc Natl Acad Sci U S A. 2014 Jun 17;111(24):8729-34. doi: 10.1073/pnas.1403294111. Epub 2014 Jun 2. Proc Natl Acad Sci U S A. 2014. PMID: 24889634 Free PMC article.
-
Interlayer water regulates the bio-nano interface of a β-sheet protein stacking on graphene.Sci Rep. 2015 Jan 5;5:7572. doi: 10.1038/srep07572. Sci Rep. 2015. PMID: 25557857 Free PMC article.
References
-
- Tanford C. The Hydrophobic Effect—Formation of Micelles and Biological Membranes. New York: Wiley Interscience; 1973.
-
- Kauzmann W. Some factors in the interpretation of protein denaturation. Adv Protein Chem. 1959;14:1–63. - PubMed
-
- Stillinger FH. Structure in aqueous solutions of nonpolar solutes from the standpoint of scaled-particle theory. J Solution Chem. 1973;2:141–158.
-
- Lum K, Chandler D, Weeks JD. Hydrophobicity at small and large length scales. J Phys Chem B. 1999;103:4570–4577.
-
- Chandler D. Interfaces and the driving force of hydrophobic assembly. Nature. 2005;437:640–647. - PubMed
Publication types
MeSH terms
Substances
Grants and funding
LinkOut - more resources
Full Text Sources
Other Literature Sources
Miscellaneous