Development of an optimized backbone of FRET biosensors for kinases and GTPases
- PMID: 21976697
- PMCID: PMC3226481
- DOI: 10.1091/mbc.E11-01-0072
Development of an optimized backbone of FRET biosensors for kinases and GTPases
Abstract
Biosensors based on the principle of Förster (or fluorescence) resonance energy transfer (FRET) have shed new light on the spatiotemporal dynamics of signaling molecules. Among them, intramolecular FRET biosensors have been increasingly used due to their high sensitivity and user-friendliness. Time-consuming optimizations by trial and error, however, obstructed the development of intramolecular FRET biosensors. Here we report an optimized backbone for rapid development of highly sensitive intramolecular FRET biosensors. The key concept is to exclude the "orientation-dependent" FRET and to render the biosensors completely "distance-dependent" with a long, flexible linker. We optimized a pair of fluorescent proteins for distance-dependent biosensors, and then developed a long, flexible linker ranging from 116 to 244 amino acids in length, which reduced the basal FRET signal and thereby increased the gain of the FRET biosensors. Computational simulations provided insight into the mechanisms by which this optimized system was the rational strategy for intramolecular FRET biosensors. With this backbone system, we improved previously reported FRET biosensors of PKA, ERK, JNK, EGFR/Abl, Ras, and Rac1. Furthermore, this backbone enabled us to develop novel FRET biosensors for several kinases of RSK, S6K, Akt, and PKC and to perform quantitative evaluation of kinase inhibitors in living cells.
Figures
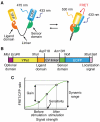
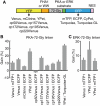
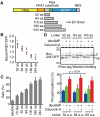
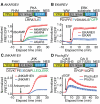
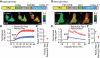
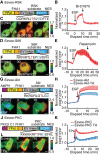
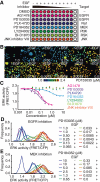
Similar articles
-
Booster, a Red-Shifted Genetically Encoded Förster Resonance Energy Transfer (FRET) Biosensor Compatible with Cyan Fluorescent Protein/Yellow Fluorescent Protein-Based FRET Biosensors and Blue Light-Responsive Optogenetic Tools.ACS Sens. 2020 Mar 27;5(3):719-730. doi: 10.1021/acssensors.9b01941. Epub 2020 Feb 26. ACS Sens. 2020. PMID: 32101394
-
Imaging of Genetically Encoded FRET-Based Biosensors to Detect GPCR Activity.Methods Mol Biol. 2021;2268:159-178. doi: 10.1007/978-1-0716-1221-7_11. Methods Mol Biol. 2021. PMID: 34085268
-
Visualization of small GTPase activity with fluorescence resonance energy transfer-based biosensors.Nat Protoc. 2009;4(11):1623-31. doi: 10.1038/nprot.2009.175. Epub 2009 Oct 15. Nat Protoc. 2009. PMID: 19834477
-
Stable expression of FRET biosensors: a new light in cancer research.Cancer Sci. 2012 Apr;103(4):614-9. doi: 10.1111/j.1349-7006.2011.02196.x. Epub 2012 Feb 3. Cancer Sci. 2012. PMID: 22188216 Free PMC article. Review.
-
Protein biosensors based on the principle of fluorescence resonance energy transfer for monitoring cellular dynamics.Biotechnol Lett. 2006 Dec;28(24):1971-82. doi: 10.1007/s10529-006-9193-5. Epub 2006 Oct 5. Biotechnol Lett. 2006. PMID: 17021660 Review.
Cited by
-
Synthetic spatially graded Rac activation drives cell polarization and movement.Proc Natl Acad Sci U S A. 2012 Dec 26;109(52):E3668-77. doi: 10.1073/pnas.1210295109. Epub 2012 Nov 26. Proc Natl Acad Sci U S A. 2012. PMID: 23185021 Free PMC article.
-
PLEKHG3 enhances polarized cell migration by activating actin filaments at the cell front.Proc Natl Acad Sci U S A. 2016 Sep 6;113(36):10091-6. doi: 10.1073/pnas.1604720113. Epub 2016 Aug 23. Proc Natl Acad Sci U S A. 2016. PMID: 27555588 Free PMC article.
-
Myocardial TRPC6-mediated Zn2+ influx induces beneficial positive inotropy through β-adrenoceptors.Nat Commun. 2022 Oct 26;13(1):6374. doi: 10.1038/s41467-022-34194-9. Nat Commun. 2022. PMID: 36289215 Free PMC article.
-
Regulation of ERK basal and pulsatile activity control proliferation and exit from the stem cell compartment in mammalian epidermis.Proc Natl Acad Sci U S A. 2020 Jul 28;117(30):17796-17807. doi: 10.1073/pnas.2006965117. Epub 2020 Jul 10. Proc Natl Acad Sci U S A. 2020. PMID: 32651268 Free PMC article.
-
ALIX Regulates Tumor-Mediated Immunosuppression by Controlling EGFR Activity and PD-L1 Presentation.Cell Rep. 2018 Jul 17;24(3):630-641. doi: 10.1016/j.celrep.2018.06.066. Cell Rep. 2018. PMID: 30021161 Free PMC article.
References
-
- Allen MD, Zhang J. Subcellular dynamics of protein kinase A activity visualized by FRET-based reporters. Biochem Biophys Res Commun. 2006;348:716–721. - PubMed
-
- Aoki K, Matsuda M. Visualization of small GTPase activity with fluorescence resonance energy transfer-based biosensors. Nat Protoc. 2009;4:1623–1631. - PubMed
-
- Chiu VK, Bivona T, Hach A, Sajous JB, Silletti J, Wiener H, Johnson RL, 2nd, Cox AD, Philips MR. Ras signalling on the endoplasmic reticulum and the Golgi. Nat Cell Biol. 2002;4:343–350. - PubMed
Publication types
MeSH terms
Substances
LinkOut - more resources
Full Text Sources
Other Literature Sources
Research Materials
Miscellaneous