A mouse model of accelerated liver aging caused by a defect in DNA repair
- PMID: 21953681
- PMCID: PMC3250572
- DOI: 10.1002/hep.24713
A mouse model of accelerated liver aging caused by a defect in DNA repair
Abstract
The liver changes with age, leading to an impaired ability to respond to hepatic insults and increased incidence of liver disease in the elderly. Therefore, there is critical need for rapid model systems to study aging-related liver changes. One potential opportunity is murine models of human progerias or diseases of accelerated aging. Ercc1(-/Δ) mice model a rare human progeroid syndrome caused by inherited defects in DNA repair. To determine whether hepatic changes that occur with normal aging occur prematurely in Ercc1(-/Δ) mice, we systematically compared liver from 5-month-old progeroid Ercc1(-/Δ) mice to old (24-36-month-old) wild-type (WT) mice. Both displayed areas of necrosis, foci of hepatocellular degeneration, and acute inflammation. Loss of hepatic architecture, fibrosis, steatosis, pseudocapillarization, and anisokaryosis were more dramatic in Ercc1(-/Δ) mice than in old WT mice. Liver enzymes were significantly elevated in serum of Ercc1(-/Δ) mice and old WT mice, whereas albumin was reduced, demonstrating liver damage and dysfunction. The regenerative capacity of Ercc1(-/Δ) liver after partial hepatectomy was significantly reduced. There was evidence of increased oxidative damage in Ercc1(-/Δ) and old WT liver, including lipofuscin, lipid hydroperoxides and acrolein, as well as increased hepatocellular senescence. There was a highly significant correlation in genome-wide transcriptional changes between old WT and 16-week-old, but not 5-week-old, Ercc1(-/Δ) mice, emphasizing that the Ercc1(-/Δ) mice acquire an aging profile in early adulthood.
Conclusion: There are strong functional, regulatory, and histopathological parallels between accelerated aging driven by a DNA repair defect and normal aging. This supports a role for DNA damage in driving aging and validates a murine model for rapidly testing hypotheses about causes and treatment for aging-related hepatic changes.
Copyright © 2011 American Association for the Study of Liver Diseases.
Figures
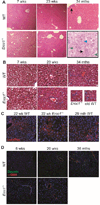
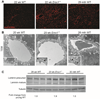
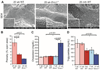
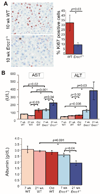
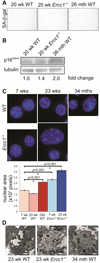
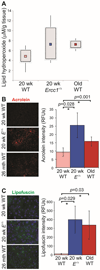
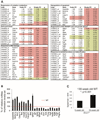
Similar articles
-
In Vivo Renin Activity Imaging in the Kidney of Progeroid Ercc1 Mutant Mice.Int J Mol Sci. 2021 Nov 18;22(22):12433. doi: 10.3390/ijms222212433. Int J Mol Sci. 2021. PMID: 34830315 Free PMC article.
-
Transcriptional profiling reveals progeroid Ercc1(-/Δ) mice as a model system for glomerular aging.BMC Genomics. 2013 Aug 16;14:559. doi: 10.1186/1471-2164-14-559. BMC Genomics. 2013. PMID: 23947592 Free PMC article.
-
mTOR signaling plays a critical role in the defects observed in muscle-derived stem/progenitor cells isolated from a murine model of accelerated aging.J Orthop Res. 2017 Jul;35(7):1375-1382. doi: 10.1002/jor.23409. Epub 2016 Sep 22. J Orthop Res. 2017. PMID: 27572850 Free PMC article.
-
Physiological consequences of defects in ERCC1-XPF DNA repair endonuclease.DNA Repair (Amst). 2011 Jul 15;10(7):781-91. doi: 10.1016/j.dnarep.2011.04.026. Epub 2011 May 25. DNA Repair (Amst). 2011. PMID: 21612988 Free PMC article. Review.
-
The ERCC1 and ERCC4 (XPF) genes and gene products.Gene. 2015 Sep 15;569(2):153-61. doi: 10.1016/j.gene.2015.06.026. Epub 2015 Jun 12. Gene. 2015. PMID: 26074087 Free PMC article. Review.
Cited by
-
NF-κB inhibition delays DNA damage-induced senescence and aging in mice.J Clin Invest. 2012 Jul;122(7):2601-12. doi: 10.1172/JCI45785. Epub 2012 Jun 18. J Clin Invest. 2012. PMID: 22706308 Free PMC article.
-
A new mouse model of radiation-induced liver disease reveals mitochondrial dysfunction as an underlying fibrotic stimulus.JHEP Rep. 2022 May 21;4(7):100508. doi: 10.1016/j.jhepr.2022.100508. eCollection 2022 Jul. JHEP Rep. 2022. PMID: 35712694 Free PMC article.
-
Sinusoidal and pericellular fibrosis in adult post-transplant liver biopsies: association with hepatic stellate cell activation and patient outcome.Virchows Arch. 2019 Aug;475(2):233-243. doi: 10.1007/s00428-019-02585-x. Epub 2019 Jun 14. Virchows Arch. 2019. PMID: 31201503 Free PMC article.
-
Chronic obstructive pulmonary disease and the hallmarks of aging.Lung India. 2018 Jul-Aug;35(4):321-327. doi: 10.4103/lungindia.lungindia_266_17. Lung India. 2018. PMID: 29970772 Free PMC article. Review.
-
Downregulation of cholesterol biosynthesis genes in the forebrain of ERCC1-deficient mice.Neurobiol Dis. 2012 Mar;45(3):1136-44. doi: 10.1016/j.nbd.2011.12.036. Epub 2011 Dec 29. Neurobiol Dis. 2012. PMID: 22245387 Free PMC article.
References
-
- Kirkwood TB. Understanding the odd science of aging. Cell. 2005;120:437–447. - PubMed
-
- Xu J, Kochanek KD, Murphy SL, Tejada-Vera B. Services USDoHaH, editor. Hyattsville, MD: 2010. Deaths: Final Data for 2007. - PubMed
-
- del Olmo JA, Serra MA, Rodriguez F, Escudero A, Gilabert S, Rodrigo JM. Incidence and risk factors for hepatocellular carcinoma in 967 patients with cirrhosis. J Cancer Res Clin Oncol. 1998;124:560–564. - PubMed
-
- Frith J, Jones D, Newton JL. Chronic liver disease in an ageing population. Age Ageing. 2009;38:11–18. - PubMed
-
- Wynne HA, Cope LH, Mutch E, Rawlins MD, Woodhouse KW, James OF. The effect of age upon liver volume and apparent liver blood flow in healthy man. Hepatology. 1989;9:297–301. - PubMed
Publication types
MeSH terms
Substances
Grants and funding
- UL1 RR024153/RR/NCRR NIH HHS/United States
- P30 DK079307/DK/NIDDK NIH HHS/United States
- R01 CA076541/CA/NCI NIH HHS/United States
- R29 CA076541/CA/NCI NIH HHS/United States
- P30 CA047904-19/CA/NCI NIH HHS/United States
- R01 ES016114-03/ES/NIEHS NIH HHS/United States
- R01 CA076541-10/CA/NCI NIH HHS/United States
- R01 ES016114/ES/NIEHS NIH HHS/United States
- R01 ES016114-05/ES/NIEHS NIH HHS/United States
- P30 DK079307-04/DK/NIDDK NIH HHS/United States
- R01 ES016114-04/ES/NIEHS NIH HHS/United States
- P30 AG024827/AG/NIA NIH HHS/United States
- P30 AG024827-05/AG/NIA NIH HHS/United States
- UL1 TR000005/TR/NCATS NIH HHS/United States
- P30DK079307/DK/NIDDK NIH HHS/United States
- ES016114/ES/NIEHS NIH HHS/United States
- P30 CA047904/CA/NCI NIH HHS/United States
- R01 ES016114-03S2/ES/NIEHS NIH HHS/United States
- P30CA047904/CA/NCI NIH HHS/United States
- P30AG024827/AG/NIA NIH HHS/United States
LinkOut - more resources
Full Text Sources
Medical