Quantification of age-dependent somatic CAG repeat instability in Hdh CAG knock-in mice reveals different expansion dynamics in striatum and liver
- PMID: 21897851
- PMCID: PMC3163641
- DOI: 10.1371/journal.pone.0023647
Quantification of age-dependent somatic CAG repeat instability in Hdh CAG knock-in mice reveals different expansion dynamics in striatum and liver
Abstract
Background: Age at onset of Huntington's disease (HD) is largely determined by the CAG trinucleotide repeat length in the HTT gene. Importantly, the CAG repeat undergoes tissue-specific somatic instability, prevalent in brain regions that are disease targets, suggesting a potential role for somatic CAG repeat instability in modifying HD pathogenesis. Thus, understanding underlying mechanisms of somatic CAG repeat instability may lead to discoveries of novel therapeutics for HD. Investigation of the dynamics of the CAG repeat size changes over time may provide insights into the mechanisms underlying CAG repeat instability.
Methodology/principal findings: To understand how the HTT CAG repeat length changes over time, we quantified somatic instability of the CAG repeat in Huntington's disease CAG knock-in mice from 2-16 months of age in liver, striatum, spleen and tail. The HTT CAG repeat in spleen and tail was very stable, but that in liver and striatum expanded over time at an average rate of one CAG per month. Interestingly, the patterns of repeat instability were different between liver and striatum. Unstable CAG repeats in liver repeatedly gained similar sizes of additional CAG repeats (approximately two CAGs per month), maintaining a distinct population of unstable repeats. In contrast, unstable CAG repeats in striatum gained additional repeats with different sizes resulting in broadly distributed unstable CAG repeats. Expanded CAG repeats in the liver were highly enriched in polyploid hepatocytes, suggesting that the pattern of liver instability may reflect the restriction of the unstable repeats to a unique cell type.
Conclusions/significance: Our results are consistent with repeat expansion occurring as a consequence of recurrent small repeat insertions that differ in different tissues. Investigation of the specific mechanisms that underlie liver and striatal instability will contribute to our understanding of the relationship between instability and disease and the means to intervene in this process.
Conflict of interest statement
Figures
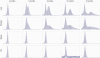
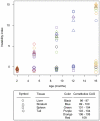
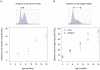
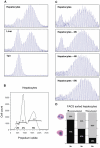
Similar articles
-
Modifiers of Somatic Repeat Instability in Mouse Models of Friedreich Ataxia and the Fragile X-Related Disorders: Implications for the Mechanism of Somatic Expansion in Huntington's Disease.J Huntingtons Dis. 2021;10(1):149-163. doi: 10.3233/JHD-200423. J Huntingtons Dis. 2021. PMID: 33579860 Free PMC article. Review.
-
Stoichiometry of base excision repair proteins correlates with increased somatic CAG instability in striatum over cerebellum in Huntington's disease transgenic mice.PLoS Genet. 2009 Dec;5(12):e1000749. doi: 10.1371/journal.pgen.1000749. Epub 2009 Dec 4. PLoS Genet. 2009. PMID: 19997493 Free PMC article.
-
Genetic background modifies nuclear mutant huntingtin accumulation and HD CAG repeat instability in Huntington's disease knock-in mice.Hum Mol Genet. 2006 Jun 15;15(12):2015-24. doi: 10.1093/hmg/ddl125. Epub 2006 May 10. Hum Mol Genet. 2006. PMID: 16687439
-
MSH3 polymorphisms and protein levels affect CAG repeat instability in Huntington's disease mice.PLoS Genet. 2013;9(2):e1003280. doi: 10.1371/journal.pgen.1003280. Epub 2013 Feb 28. PLoS Genet. 2013. PMID: 23468640 Free PMC article.
-
Methods for Assessing DNA Repair and Repeat Expansion in Huntington's Disease.Methods Mol Biol. 2018;1780:483-495. doi: 10.1007/978-1-4939-7825-0_22. Methods Mol Biol. 2018. PMID: 29856032 Review.
Cited by
-
Transcriptional correlates of the pathological phenotype in a Huntington's disease mouse model.Sci Rep. 2019 Dec 10;9(1):18696. doi: 10.1038/s41598-019-55177-9. Sci Rep. 2019. PMID: 31822756 Free PMC article.
-
Modifiers of Somatic Repeat Instability in Mouse Models of Friedreich Ataxia and the Fragile X-Related Disorders: Implications for the Mechanism of Somatic Expansion in Huntington's Disease.J Huntingtons Dis. 2021;10(1):149-163. doi: 10.3233/JHD-200423. J Huntingtons Dis. 2021. PMID: 33579860 Free PMC article. Review.
-
Huntington's disease and the striatal medium spiny neuron: cell-autonomous and non-cell-autonomous mechanisms of disease.Neurotherapeutics. 2012 Apr;9(2):270-84. doi: 10.1007/s13311-012-0112-2. Neurotherapeutics. 2012. PMID: 22441874 Free PMC article. Review.
-
HttQ111/+ Huntington's Disease Knock-in Mice Exhibit Brain Region-Specific Morphological Changes and Synaptic Dysfunction.J Huntingtons Dis. 2018;7(1):17-33. doi: 10.3233/JHD-170282. J Huntingtons Dis. 2018. PMID: 29480209 Free PMC article.
-
The Repeat Expansion Diseases: The dark side of DNA repair.DNA Repair (Amst). 2015 Aug;32:96-105. doi: 10.1016/j.dnarep.2015.04.019. Epub 2015 Apr 30. DNA Repair (Amst). 2015. PMID: 26002199 Free PMC article. Review.
References
-
- Huntington G. On chorea. Med Surg Rep. 1872;26:320–321.
-
- Huntington's disease collaborative research group. A novel gene containing a trinucleotide repeat that is expanded and unstable on Huntington's disease chromosomes. The Huntington's Disease Collaborative Research Group. Cell. 1993;72:971–983. - PubMed
-
- Andrew SE, Goldberg YP, Kremer B, Telenius H, Theilmann J, et al. The relationship between trinucleotide (CAG) repeat length and clinical features of Huntington's disease. Nat Genet. 1993;4:398–403. - PubMed
-
- Duyao M, Ambrose C, Myers R, Novelletto A, Persichetti F, et al. Trinucleotide repeat length instability and age of onset in Huntington's disease. Nat Genet. 1993;4:387–392. - PubMed
Publication types
MeSH terms
Substances
Grants and funding
LinkOut - more resources
Full Text Sources
Other Literature Sources
Medical
Molecular Biology Databases