Control of excitatory CNS synaptogenesis by astrocyte-secreted proteins Hevin and SPARC
- PMID: 21788491
- PMCID: PMC3156217
- DOI: 10.1073/pnas.1104977108
Control of excitatory CNS synaptogenesis by astrocyte-secreted proteins Hevin and SPARC
Abstract
Astrocytes regulate synaptic connectivity in the CNS through secreted signals. Here we identified two astrocyte-secreted proteins, hevin and SPARC, as regulators of excitatory synaptogenesis in vitro and in vivo. Hevin induces the formation of synapses between cultured rat retinal ganglion cells. SPARC is not synaptogenic, but specifically antagonizes synaptogenic function of hevin. Hevin and SPARC are expressed by astrocytes in the superior colliculus, the synaptic target of retinal ganglion cells, concurrent with the excitatory synaptogenesis. Hevin-null mice had fewer excitatory synapses; conversely, SPARC-null mice had increased synaptic connections in the superior colliculus. Furthermore, we found that hevin is required for the structural maturation of the retinocollicular synapses. These results identify hevin as a positive and SPARC as a negative regulator of synapse formation and signify that, through regulation of relative levels of hevin and SPARC, astrocytes might control the formation, maturation, and plasticity of synapses in vivo.
Conflict of interest statement
The authors declare no conflict of interest.
Figures
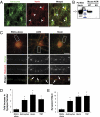
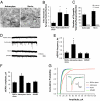
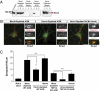
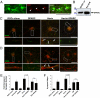
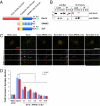
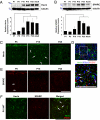
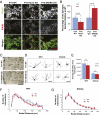
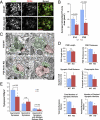
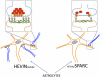
Similar articles
-
Interplay between hevin, SPARC, and MDGAs: Modulators of neurexin-neuroligin transsynaptic bridges.Structure. 2021 Jul 1;29(7):664-678.e6. doi: 10.1016/j.str.2021.01.003. Epub 2021 Feb 2. Structure. 2021. PMID: 33535026 Free PMC article.
-
Heterogeneity in Synaptogenic Profile of Astrocytes from Different Brain Regions.Mol Neurobiol. 2018 Jan;55(1):751-762. doi: 10.1007/s12035-016-0343-z. Epub 2017 Jan 3. Mol Neurobiol. 2018. PMID: 28050794
-
Roles of the synaptic molecules Hevin and SPARC in mouse neuromuscular junction development and repair.Neurosci Lett. 2021 Feb 16;746:135663. doi: 10.1016/j.neulet.2021.135663. Epub 2021 Jan 22. Neurosci Lett. 2021. PMID: 33493647 Free PMC article.
-
Astrocyte-secreted matricellular proteins in CNS remodelling during development and disease.Neural Plast. 2014;2014:321209. doi: 10.1155/2014/321209. Epub 2014 Jan 16. Neural Plast. 2014. PMID: 24551460 Free PMC article. Review.
-
Hevin/SC1, a matricellular glycoprotein and potential tumor-suppressor of the SPARC/BM-40/Osteonectin family.Int J Biochem Cell Biol. 2004 Jun;36(6):991-6. doi: 10.1016/j.biocel.2004.01.017. Int J Biochem Cell Biol. 2004. PMID: 15094114 Review.
Cited by
-
Glial cell type-specific gene expression in the mouse cerebrum using the piggyBac system and in utero electroporation.Sci Rep. 2021 Mar 1;11(1):4864. doi: 10.1038/s41598-021-84210-z. Sci Rep. 2021. PMID: 33649472 Free PMC article.
-
Cloning, characterization, and spatio-temporal expression patterns of HdhSPARC and its responses to multiple stressors.Sci Rep. 2024 Jan 26;14(1):2224. doi: 10.1038/s41598-024-51950-7. Sci Rep. 2024. PMID: 38278828 Free PMC article.
-
Emerging roles of astrocytes in neural circuit development.Nat Rev Neurosci. 2013 May;14(5):311-21. doi: 10.1038/nrn3484. Epub 2013 Apr 18. Nat Rev Neurosci. 2013. PMID: 23595014 Free PMC article. Review.
-
Ablation of reactive astrocytes exacerbates disease pathology in a model of Alzheimer's disease.Glia. 2020 May;68(5):1017-1030. doi: 10.1002/glia.23759. Epub 2019 Dec 4. Glia. 2020. PMID: 31799735 Free PMC article.
-
Interaction of Nerve Growth Factor β with Adiponectin and SPARC Oppositely Modulates its Biological Activity.Int J Mol Sci. 2019 Mar 27;20(7):1541. doi: 10.3390/ijms20071541. Int J Mol Sci. 2019. PMID: 30934765 Free PMC article.
References
-
- Bolton MM, Eroglu C. Look who is weaving the neural web: Glial control of synapse formation. Curr Opin Neurobiol. 2009;19:491–497. - PubMed
-
- Meyer-Franke A, Kaplan MR, Pfrieger FW, Barres BA. Characterization of the signaling interactions that promote the survival and growth of developing retinal ganglion cells in culture. Neuron. 1995;15:805–819. - PubMed
-
- Christopherson KS, et al. Thrombospondins are astrocyte-secreted proteins that promote CNS synaptogenesis. Cell. 2005;120:421–433. - PubMed
Publication types
MeSH terms
Substances
Grants and funding
LinkOut - more resources
Full Text Sources
Other Literature Sources
Molecular Biology Databases
Miscellaneous