Streamlined platform for short hairpin RNA interference and transgenesis in cultured mammalian cells
- PMID: 21768390
- PMCID: PMC3150880
- DOI: 10.1073/pnas.1103532108
Streamlined platform for short hairpin RNA interference and transgenesis in cultured mammalian cells
Abstract
Sequence-specific gene silencing by short hairpin (sh) RNAs has recently emerged as an indispensable tool for understanding gene function and a promising avenue for drug discovery. However, a wider biomedical use of this approach is hindered by the lack of straightforward methods for achieving uniform expression of shRNAs in mammalian cell cultures. Here we report a high-efficiency and low-background (HILO) recombination-mediated cassette exchange (RMCE) technology that yields virtually homogeneous cell pools containing doxycycline-inducible shRNA elements in a matter of days and with minimal efforts. To ensure immediate utility of this approach for a wider research community, we modified 11 commonly used human (A549, HT1080, HEK293T, HeLa, HeLa-S3, and U2OS) and mouse (CAD, L929, N2a, NIH 3T3, and P19) cell lines to be compatible with the HILO-RMCE process. Because of its technical simplicity and cost efficiency, the technology will be advantageous for both low- and high-throughput shRNA experiments. We also provide evidence that HILO-RMCE will facilitate a wider range of molecular and cell biology applications by allowing one to rapidly engineer cell populations expressing essentially any transgene of interest.
Conflict of interest statement
The authors declare no conflict of interest.
Figures
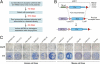
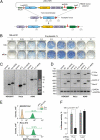
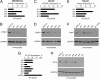
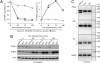
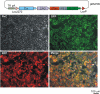
Similar articles
-
DNA vector-based RNA interference to study gene function in cancer.J Vis Exp. 2012 Jun 4;(64):e4129. doi: 10.3791/4129. J Vis Exp. 2012. PMID: 22710444 Free PMC article.
-
Conditional gene expression and knockdown using lentivirus vectors encoding shRNA.Methods Mol Biol. 2008;434:291-309. doi: 10.1007/978-1-60327-248-3_18. Methods Mol Biol. 2008. PMID: 18470652
-
Targeting nuclear receptors with lentivirus-delivered small RNAs in primary human hepatocytes.Cell Physiol Biochem. 2014;33(6):2003-13. doi: 10.1159/000362976. Cell Physiol Biochem. 2014. PMID: 25012546
-
Optimization of short hairpin RNA for lentiviral-mediated RNAi against WAS.Biochem Biophys Res Commun. 2007 Oct 19;362(2):498-503. doi: 10.1016/j.bbrc.2007.08.013. Epub 2007 Aug 10. Biochem Biophys Res Commun. 2007. PMID: 17719003
-
Mammalian RNAi: a practical guide.Biotechniques. 2005 Aug;39(2):215-24. doi: 10.2144/05392RV01. Biotechniques. 2005. PMID: 16116795 Review.
Cited by
-
CRISPR Cas13-Based Tools to Track and Manipulate Endogenous Telomeric Repeat-Containing RNAs in Live Cells.Front Mol Biosci. 2022 Jan 31;8:785160. doi: 10.3389/fmolb.2021.785160. eCollection 2021. Front Mol Biosci. 2022. PMID: 35174207 Free PMC article.
-
RNA-binding protein HuR autoregulates its expression by promoting alternative polyadenylation site usage.Nucleic Acids Res. 2012 Jan;40(2):787-800. doi: 10.1093/nar/gkr783. Epub 2011 Sep 24. Nucleic Acids Res. 2012. PMID: 21948791 Free PMC article.
-
Localized light-induced protein dimerization in living cells using a photocaged dimerizer.Nat Commun. 2014 Nov 17;5:5475. doi: 10.1038/ncomms6475. Nat Commun. 2014. PMID: 25400104 Free PMC article.
-
LABRAT reveals association of alternative polyadenylation with transcript localization, RNA binding protein expression, transcription speed, and cancer survival.BMC Genomics. 2021 Jun 26;22(1):476. doi: 10.1186/s12864-021-07781-1. BMC Genomics. 2021. PMID: 34174817 Free PMC article.
-
Alternative splicing coupled to nonsense-mediated decay coordinates downregulation of non-neuronal genes in developing mouse neurons.Genome Biol. 2024 Jun 20;25(1):162. doi: 10.1186/s13059-024-03305-8. Genome Biol. 2024. PMID: 38902825 Free PMC article.
References
-
- Fire A, et al. Potent and specific genetic interference by double-stranded RNA in Caenorhabditis elegans. Nature. 1998;391:806–811. - PubMed
-
- Hannon GJ, Rossi JJ. Unlocking the potential of the human genome with RNA interference. Nature. 2004;431:371–378. - PubMed
-
- Kim VN, Han J, Siomi MC. Biogenesis of small RNAs in animals. Nat Rev Mol Cell Biol. 2009;10(2):126–139. - PubMed
Publication types
MeSH terms
Substances
LinkOut - more resources
Full Text Sources
Other Literature Sources
Molecular Biology Databases
Miscellaneous