Deletion of the monkeypox virus inhibitor of complement enzymes locus impacts the adaptive immune response to monkeypox virus in a nonhuman primate model of infection
- PMID: 21752919
- PMCID: PMC3165757
- DOI: 10.1128/JVI.00199-11
Deletion of the monkeypox virus inhibitor of complement enzymes locus impacts the adaptive immune response to monkeypox virus in a nonhuman primate model of infection
Abstract
Monkeypox virus (MPXV) is an orthopoxvirus closely related to variola virus, the causative agent of smallpox. Human MPXV infection results in a disease that is similar to smallpox and can also be fatal. Two clades of MPXV have been identified, with viruses of the central African clade displaying more pathogenic properties than those within the west African clade. The monkeypox inhibitor of complement enzymes (MOPICE), which is not expressed by viruses of the west African clade, has been hypothesized to be a main virulence factor responsible for increased pathogenic properties of central African strains of MPXV. To gain a better understanding of the role of MOPICE during MPXV-mediated disease, we compared the host adaptive immune response and disease severity following intrabronchial infection with MPXV-Zaire (n = 4), or a recombinant MPXV-Zaire (n = 4) lacking expression of MOPICE in rhesus macaques (RM). Data presented here demonstrate that infection of RM with MPXV leads to significant viral replication in the peripheral blood and lungs and results in the induction of a robust and sustained adaptive immune response against the virus. More importantly, we show that the loss of MOPICE expression results in enhanced viral replication in vivo, as well as a dampened adaptive immune response against MPXV. Taken together, these findings suggest that MOPICE modulates the anti-MPXV immune response and that this protein is not the sole virulence factor of the central African clade of MPXV.
Figures
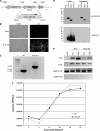
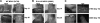
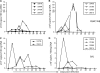
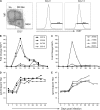
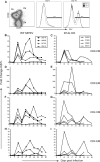
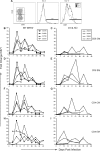
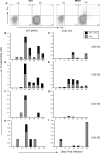
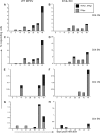
Similar articles
-
Elucidating the role of the complement control protein in monkeypox pathogenicity.PLoS One. 2012;7(4):e35086. doi: 10.1371/journal.pone.0035086. Epub 2012 Apr 9. PLoS One. 2012. PMID: 22496894 Free PMC article.
-
Attenuated NYCBH vaccinia virus deleted for the E3L gene confers partial protection against lethal monkeypox virus disease in cynomolgus macaques.Vaccine. 2011 Dec 6;29(52):9684-90. doi: 10.1016/j.vaccine.2011.09.135. Epub 2011 Oct 12. Vaccine. 2011. PMID: 22001879 Free PMC article.
-
Molecular Virology of Orthopoxviruses with Special Reference to Monkeypox Virus.Adv Exp Med Biol. 2024;1451:111-124. doi: 10.1007/978-3-031-57165-7_7. Adv Exp Med Biol. 2024. PMID: 38801574 Review.
-
The highly virulent variola and monkeypox viruses express secreted inhibitors of type I interferon.FASEB J. 2010 May;24(5):1479-88. doi: 10.1096/fj.09-144733. Epub 2009 Dec 17. FASEB J. 2010. PMID: 20019241 Free PMC article.
-
Mpox global health emergency: Insights into the virus, immune responses, and advancements in vaccines PART I: Insights into the virus and immune responses.Asian Pac J Allergy Immunol. 2024 Sep;42(3):181-190. doi: 10.12932/AP-111024-1945. Asian Pac J Allergy Immunol. 2024. PMID: 39417767 Review.
Cited by
-
Monkeypox: disease epidemiology, host immunity and clinical interventions.Nat Rev Immunol. 2022 Oct;22(10):597-613. doi: 10.1038/s41577-022-00775-4. Epub 2022 Sep 5. Nat Rev Immunol. 2022. PMID: 36064780 Free PMC article. Review.
-
Monkeypox: The Re-emerging Terror.Cureus. 2022 Aug 30;14(8):e28597. doi: 10.7759/cureus.28597. eCollection 2022 Aug. Cureus. 2022. PMID: 36185856 Free PMC article. Review.
-
Attenuation of monkeypox virus by deletion of genomic regions.Virology. 2015 Jan 15;475:129-38. doi: 10.1016/j.virol.2014.11.009. Epub 2014 Dec 1. Virology. 2015. PMID: 25462353 Free PMC article.
-
An overview on monkeypox virus: Pathogenesis, transmission, host interaction and therapeutics.Front Cell Infect Microbiol. 2023 Feb 10;13:1076251. doi: 10.3389/fcimb.2023.1076251. eCollection 2023. Front Cell Infect Microbiol. 2023. PMID: 36844409 Free PMC article. Review.
-
T cell inactivation by poxviral B22 family proteins increases viral virulence.PLoS Pathog. 2014 May 15;10(5):e1004123. doi: 10.1371/journal.ppat.1004123. eCollection 2014 May. PLoS Pathog. 2014. PMID: 24832205 Free PMC article.
References
-
- Amanna I. J., Slifka M. K., Crotty S. 2006. Immunity and immunological memory following smallpox vaccination. Immunol. Rev. 211:320–337 - PubMed
-
- Cameron C. M., Barrett J. W., Mann M., Lucas A., McFadden G. 2005. Myxoma virus M128L is expressed as a cell surface CD47-like virulence factor that contributes to the downregulation of macrophage activation in vivo. Virology 337:55–67 - PubMed
Publication types
MeSH terms
Substances
Associated data
- Actions
- Actions
Grants and funding
LinkOut - more resources
Full Text Sources