Gut feelings: the emerging biology of gut-brain communication
- PMID: 21750565
- PMCID: PMC3845678
- DOI: 10.1038/nrn3071
Gut feelings: the emerging biology of gut-brain communication
Abstract
The concept that the gut and the brain are closely connected, and that this interaction plays an important part not only in gastrointestinal function but also in certain feeling states and in intuitive decision making, is deeply rooted in our language. Recent neurobiological insights into this gut-brain crosstalk have revealed a complex, bidirectional communication system that not only ensures the proper maintenance of gastrointestinal homeostasis and digestion but is likely to have multiple effects on affect, motivation and higher cognitive functions, including intuitive decision making. Moreover, disturbances of this system have been implicated in a wide range of disorders, including functional and inflammatory gastrointestinal disorders, obesity and eating disorders.
Figures
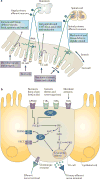
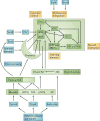
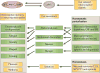
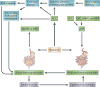
Similar articles
-
Mind-altering with the gut: Modulation of the gut-brain axis with probiotics.J Microbiol. 2018 Mar;56(3):172-182. doi: 10.1007/s12275-018-8032-4. Epub 2018 Feb 28. J Microbiol. 2018. PMID: 29492874 Review.
-
Digestion. Gastrointestinal nutrient chemosensing and the gut-brain axis. Preface.Digestion. 2011;83 Suppl 1:IV. doi: 10.1159/000323802. Epub 2011 Mar 9. Digestion. 2011. PMID: 21389720 No abstract available.
-
Maintenance of Gastrointestinal Glucose Homeostasis by the Gut-Brain Axis.Curr Protein Pept Sci. 2017;18(6):541-547. doi: 10.2174/1389203717666160627083604. Curr Protein Pept Sci. 2017. PMID: 27356933 Review.
-
The brain-gut axis in health and disease.Adv Exp Med Biol. 2014;817:135-53. doi: 10.1007/978-1-4939-0897-4_6. Adv Exp Med Biol. 2014. PMID: 24997032 Review.
-
Aging of the mammalian gastrointestinal tract: a complex organ system.Age (Dordr). 2014 Jun;36(3):9603. doi: 10.1007/s11357-013-9603-2. Epub 2013 Dec 20. Age (Dordr). 2014. PMID: 24352567 Free PMC article. Review.
Cited by
-
Association of Loneliness and Wisdom With Gut Microbial Diversity and Composition: An Exploratory Study.Front Psychiatry. 2021 Mar 25;12:648475. doi: 10.3389/fpsyt.2021.648475. eCollection 2021. Front Psychiatry. 2021. PMID: 33841213 Free PMC article.
-
The intestinal microbiota as an ally in the treatment of Alzheimer's disease.Gut Microbiome (Camb). 2023 Jun 19;4:e9. doi: 10.1017/gmb.2023.8. eCollection 2023. Gut Microbiome (Camb). 2023. PMID: 39295910 Free PMC article. Review.
-
The role of probiotics on the roadmap to a healthy microbiota: a symposium report.Gut Microbiome (Camb). 2020 Aug 26;1:e2. doi: 10.1017/gmb.2020.2. eCollection 2020. Gut Microbiome (Camb). 2020. PMID: 39296722 Free PMC article. Review.
-
Anxiolytic effects of a galacto-oligosaccharides prebiotic in healthy females (18-25 years) with corresponding changes in gut bacterial composition.Sci Rep. 2021 Apr 15;11(1):8302. doi: 10.1038/s41598-021-87865-w. Sci Rep. 2021. PMID: 33859330 Free PMC article.
-
Gut-Brain Axis: Role of Gut Microbiota on Neurological Disorders and How Probiotics/Prebiotics Beneficially Modulate Microbial and Immune Pathways to Improve Brain Functions.Int J Mol Sci. 2020 Oct 13;21(20):7551. doi: 10.3390/ijms21207551. Int J Mol Sci. 2020. PMID: 33066156 Free PMC article. Review.
References
-
- Furness JB. The Enteric Nervous System. Blackwell; Oxford: 2006. A comprehensive overview of all aspects of the enteric nervous system.
-
- Furness JB, Kunze WA, Bertrand PP, Clerc N, Bornstein JC. Intrinsic primary afferent neurons of the intestine. Prog Neurobiol. 1998;54:1–18. - PubMed
-
- Costa M, Furness JB, Llewellyn-Smith IJ, Johnson LR. In: Physiology of the Gastrointestinal Tract. Johnson LR, et al., editors. Raven; New York: 1987. pp. 1–40.
-
- Gershon MD, et al. In: Physiology of the Gastrointestinal Tract. Johnson LR, et al., editors. Raven Press; New York: 1994. pp. 381–422.
-
- Gershon MD. The Second Brain. Harper Collins; New York: 1998.
Publication types
MeSH terms
Grants and funding
LinkOut - more resources
Full Text Sources
Other Literature Sources