Differential regulation of cellulose orientation at the inner and outer face of epidermal cells in the Arabidopsis hypocotyl
- PMID: 21742992
- PMCID: PMC3226210
- DOI: 10.1105/tpc.111.087338
Differential regulation of cellulose orientation at the inner and outer face of epidermal cells in the Arabidopsis hypocotyl
Abstract
It is generally believed that cell elongation is regulated by cortical microtubules, which guide the movement of cellulose synthase complexes as they secrete cellulose microfibrils into the periplasmic space. Transversely oriented microtubules are predicted to direct the deposition of a parallel array of microfibrils, thus generating a mechanically anisotropic cell wall that will favor elongation and prevent radial swelling. Thus far, support for this model has been most convincingly demonstrated in filamentous algae. We found that in etiolated Arabidopsis thaliana hypocotyls, microtubules and cellulose synthase trajectories are transversely oriented on the outer surface of the epidermis for only a short period during growth and that anisotropic growth continues after this transverse organization is lost. Our data support previous findings that the outer epidermal wall is polylamellate in structure, with little or no anisotropy. By contrast, we observed perfectly transverse microtubules and microfibrils at the inner face of the epidermis during all stages of cell expansion. Experimental perturbation of cortical microtubule organization preferentially at the inner face led to increased radial swelling. Our study highlights the previously underestimated complexity of cortical microtubule organization in the shoot epidermis and underscores a role for the inner tissues in the regulation of growth anisotropy.
Figures
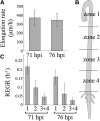
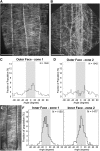
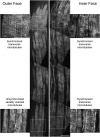
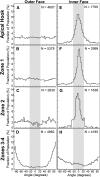
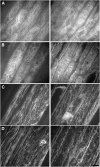
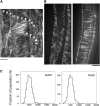
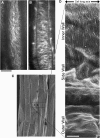
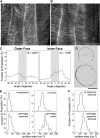
Similar articles
-
Microtubules and CESA tracks at the inner epidermal wall align independently of those on the outer wall of light-grown Arabidopsis hypocotyls.J Cell Sci. 2011 Apr 1;124(Pt 7):1088-94. doi: 10.1242/jcs.086702. Epub 2011 Mar 1. J Cell Sci. 2011. PMID: 21363888
-
Alteration of oriented deposition of cellulose microfibrils by mutation of a katanin-like microtubule-severing protein.Plant Cell. 2002 Sep;14(9):2145-60. doi: 10.1105/tpc.003947. Plant Cell. 2002. PMID: 12215512 Free PMC article.
-
Cortical microtubules optimize cell-wall crystallinity to drive unidirectional growth in Arabidopsis.Plant J. 2011 Jun;66(6):915-28. doi: 10.1111/j.1365-313X.2011.04552.x. Epub 2011 Apr 28. Plant J. 2011. PMID: 21535258
-
Cortical microtubule arrays in the Arabidopsis seedling.Curr Opin Plant Biol. 2008 Feb;11(1):94-8. doi: 10.1016/j.pbi.2007.12.001. Epub 2008 Jan 15. Curr Opin Plant Biol. 2008. PMID: 18226578 Review.
-
Establishing and maintaining axial growth: wall mechanical properties and the cytoskeleton.J Plant Res. 2006 Jan;119(1):5-10. doi: 10.1007/s10265-005-0233-3. Epub 2005 Nov 12. J Plant Res. 2006. PMID: 16284708 Review.
Cited by
-
Multiscale models in the biomechanics of plant growth.Physiology (Bethesda). 2015 Mar;30(2):159-66. doi: 10.1152/physiol.00030.2014. Physiology (Bethesda). 2015. PMID: 25729061 Free PMC article. Review.
-
A cellulose synthesis inhibitor affects cellulose synthase complex secretion and cortical microtubule dynamics.Plant Physiol. 2024 Sep 2;196(1):124-136. doi: 10.1093/plphys/kiae232. Plant Physiol. 2024. PMID: 38833284 Free PMC article.
-
Diffuse Growth of Plant Cell Walls.Plant Physiol. 2018 Jan;176(1):16-27. doi: 10.1104/pp.17.01541. Epub 2017 Nov 14. Plant Physiol. 2018. PMID: 29138349 Free PMC article. Review.
-
Mechanically, the Shoot Apical Meristem of Arabidopsis Behaves like a Shell Inflated by a Pressure of About 1 MPa.Front Plant Sci. 2015 Nov 26;6:1038. doi: 10.3389/fpls.2015.01038. eCollection 2015. Front Plant Sci. 2015. PMID: 26635855 Free PMC article.
-
FASCICLIN-LIKE 18 Is a New Player Regulating Root Elongation in Arabidopsis thaliana.Front Plant Sci. 2021 Apr 7;12:645286. doi: 10.3389/fpls.2021.645286. eCollection 2021. Front Plant Sci. 2021. PMID: 33897736 Free PMC article.
References
-
- Abe H., Funada R., Ohtani J., Fukazawa K. (1995). Changes in the arrangement of microtubules and microfibrils in differentiating conifer tracheids during the expansion of cells. Ann. Bot. (Lond.) 75: 305–310
-
- Barton D.A., Overall R.L. (2010). Cryofixation rapidly preserves cytoskeletal arrays of leaf epidermal cells revealing microtubule co-alignments between neighbouring cells and adjacent actin and microtubule bundles in the cortex. J. Microsc. 237: 79–88 - PubMed
-
- Baskin T.I. (2001). On the alignment of cellulose microfibrils by cortical microtubules: A review and a model. Protoplasma 215: 150–171 - PubMed
-
- Baskin T.I. (2005). Anisotropic expansion of the plant cell wall. Annu. Rev. Cell Dev. Biol. 21: 203–222 - PubMed
Publication types
MeSH terms
Substances
LinkOut - more resources
Full Text Sources
Other Literature Sources