Effect of Src kinase phosphorylation on disordered C-terminal domain of N-methyl-D-aspartic acid (NMDA) receptor subunit GluN2B protein
- PMID: 21712388
- PMCID: PMC3191031
- DOI: 10.1074/jbc.M111.258897
Effect of Src kinase phosphorylation on disordered C-terminal domain of N-methyl-D-aspartic acid (NMDA) receptor subunit GluN2B protein
Abstract
NMDA receptors are ligand-gated ion channels with a regulatory intracellular C-terminal domain (CTD). In GluN2B, the CTD is the largest domain in the protein but is intrinsically disordered. The GluN2B subunit is the major tyrosine-phosphorylated protein in synapses. Src kinase phosphorylates the GluN2B CTD, but it is unknown how this affects channel activity. In disordered proteins, phosphorylation can tip the balance between order and disorder. Transitions can occur in both directions, so it is not currently possible to predict the effects of phosphorylation. We used single molecule fluorescence to characterize the effects of Src phosphorylation on GluN2B. Scanning fluorescent labeling sites throughout the domain showed no positional dependence of the energy transfer. Instead, efficiency only scaled with the separation between labeling sites suggestive of a relatively featureless conformational energy landscape. Src phosphorylation led to a general expansion of the polypeptide, which would result in greater exposure of known protein-binding sites and increase the physical separation between contiguous sites. Phosphorylation makes the CTD more like a random coil leaving open the question of how Src exerts its effects on the NMDA receptor.
Figures
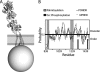
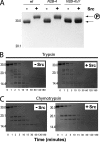
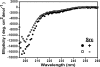
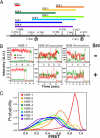
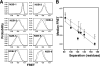
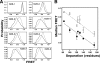
Similar articles
-
Tyrosine phosphorylation of the N-methyl-D-aspartate receptor by exogenous and postsynaptic density-associated Src-family kinases.J Neurochem. 2001 Aug;78(3):524-34. doi: 10.1046/j.1471-4159.2001.00433.x. J Neurochem. 2001. PMID: 11483655
-
Dopamine promotes NMDA receptor hypofunction in the retina through D1 receptor-mediated Csk activation, Src inhibition and decrease of GluN2B phosphorylation.Sci Rep. 2017 Jan 18;7:40912. doi: 10.1038/srep40912. Sci Rep. 2017. PMID: 28098256 Free PMC article.
-
NMDA channel regulation by channel-associated protein tyrosine kinase Src.Science. 1997 Jan 31;275(5300):674-8. doi: 10.1126/science.275.5300.674. Science. 1997. PMID: 9005855
-
Regulation of NMDA receptors by the tyrosine kinase Fyn.FEBS J. 2012 Jan;279(1):12-9. doi: 10.1111/j.1742-4658.2011.08391.x. Epub 2011 Dec 5. FEBS J. 2012. PMID: 21985328 Review.
-
The regulation of N-methyl-D-aspartate receptors by Src kinase.FEBS J. 2012 Jan;279(1):20-8. doi: 10.1111/j.1742-4658.2011.08413.x. Epub 2011 Dec 5. FEBS J. 2012. PMID: 22060915 Review.
Cited by
-
Single-molecule FRET reveals the native-state dynamics of the IκBα ankyrin repeat domain.J Mol Biol. 2013 Jul 24;425(14):2578-90. doi: 10.1016/j.jmb.2013.04.015. Epub 2013 Apr 22. J Mol Biol. 2013. PMID: 23619335 Free PMC article.
-
NMDA receptor C-terminal signaling in development, plasticity, and disease.F1000Res. 2019 Aug 30;8:F1000 Faculty Rev-1547. doi: 10.12688/f1000research.19925.1. eCollection 2019. F1000Res. 2019. PMID: 31508206 Free PMC article. Review.
-
Cancer/testis antigen PAGE4, a regulator of c-Jun transactivation, is phosphorylated by homeodomain-interacting protein kinase 1, a component of the stress-response pathway.Biochemistry. 2014 Mar 18;53(10):1670-9. doi: 10.1021/bi500013w. Epub 2014 Mar 5. Biochemistry. 2014. PMID: 24559171 Free PMC article.
-
Src Family Kinases in Brain Edema After Acute Brain Injury.Acta Neurochir Suppl. 2016;121:185-90. doi: 10.1007/978-3-319-18497-5_33. Acta Neurochir Suppl. 2016. PMID: 26463946 Free PMC article.
-
Conformational change of syntaxin linker region induced by Munc13s initiates SNARE complex formation in synaptic exocytosis.EMBO J. 2017 Mar 15;36(6):816-829. doi: 10.15252/embj.201695775. Epub 2017 Jan 30. EMBO J. 2017. PMID: 28137749 Free PMC article.
References
Publication types
MeSH terms
Substances
Grants and funding
LinkOut - more resources
Full Text Sources
Other Literature Sources
Miscellaneous