Digging deeper into lymphatic vessel formation in vitro and in vivo
- PMID: 21702933
- PMCID: PMC3141733
- DOI: 10.1186/1471-2121-12-29
Digging deeper into lymphatic vessel formation in vitro and in vivo
Abstract
Background: Abnormal lymphatic vessel formation (lymphangiogenesis) is associated with different pathologies such as cancer, lymphedema, psoriasis and graft rejection. Lymphatic vasculature displays distinctive features than blood vasculature, and mechanisms underlying the formation of new lymphatic vessels during physiological and pathological processes are still poorly documented. Most studies on lymphatic vessel formation are focused on organism development rather than lymphangiogenic events occurring in adults. We have here studied lymphatic vessel formation in two in vivo models of pathological lymphangiogenesis (corneal assay and lymphangioma). These data have been confronted to those generated in the recently set up in vitro model of lymphatic ring assay. Ultrastructural analyses through Transmission Electron Microscopy (TEM) were performed to investigate tube morphogenesis, an important differentiating process observed during endothelial cell organization into capillary structures.
Results: In both in vivo models (lymphangiogenic corneal assay and lymphangioma), migrating lymphatic endothelial cells extended long processes exploring the neighboring environment and organized into cord-like structures. Signs of intense extracellular matrix remodeling were observed extracellularly and inside cytoplasmic vacuoles. The formation of intercellular spaces between endothelial cells led to tube formation. Proliferating lymphatic endothelial cells were detected both at the tips of sprouting capillaries and inside extending sprouts. The different steps of lymphangiogenesis observed in vivo are fully recapitulated in vitro, in the lymphatic ring assay and include: (1) endothelial cell alignment in cord like structure, (2) intracellular vacuole formation and (3) matrix degradation.
Conclusions: In this study, we are providing evidence for lymphatic vessel formation through tunneling relying on extensive matrix remodeling, migration and alignment of sprouting endothelial cells into tubular structures. In addition, our data emphasize the suitability of the lymphatic ring assay to unravel mechanisms underlying lymphangiogenesis.
Figures
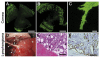
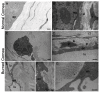
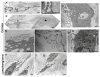
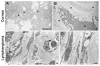
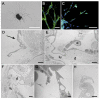
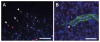
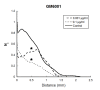
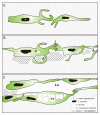
Similar articles
-
Multiple expressions of lymphatic markers and morphological evolution of newly formed lymphatics in lymphangioma and lymph node lymphangiogenesis.Microvasc Res. 2010 Sep;80(2):195-201. doi: 10.1016/j.mvr.2010.04.002. Epub 2010 Apr 9. Microvasc Res. 2010. PMID: 20382171
-
Lymphangiogenesis: in vitro and in vivo models.FASEB J. 2010 Jan;24(1):8-21. doi: 10.1096/fj.09-132852. Epub 2009 Sep 2. FASEB J. 2010. PMID: 19726757 Review.
-
Paralemmin-1 is expressed in lymphatic endothelial cells and modulates cell migration, cell maturation and tumor lymphangiogenesis.Angiogenesis. 2013 Oct;16(4):795-807. doi: 10.1007/s10456-013-9356-7. Epub 2013 May 26. Angiogenesis. 2013. PMID: 23709172
-
Polydom Is an Extracellular Matrix Protein Involved in Lymphatic Vessel Remodeling.Circ Res. 2017 Apr 14;120(8):1276-1288. doi: 10.1161/CIRCRESAHA.116.308825. Epub 2017 Feb 8. Circ Res. 2017. PMID: 28179430
-
Modeling lymphangiogenesis: Pairing in vitro and in vivo metrics.Microcirculation. 2023 Apr;30(2-3):e12802. doi: 10.1111/micc.12802. Epub 2023 Feb 28. Microcirculation. 2023. PMID: 36760223 Free PMC article. Review.
Cited by
-
Lymphangiogenesis in non-Hodgkin's lymphoma and its correlation with cyclooxygenase-2 and vascular endothelial growth factor-C.Oncol Lett. 2012 Oct;4(4):695-700. doi: 10.3892/ol.2012.817. Epub 2012 Jul 23. Oncol Lett. 2012. PMID: 23205085 Free PMC article.
-
Matrix stiffness primes lymphatic tube formation directed by vascular endothelial growth factor-C.FASEB J. 2021 May;35(5):e21498. doi: 10.1096/fj.202002426RR. FASEB J. 2021. PMID: 33774872 Free PMC article.
-
Small bowel lymphangioma causing ileo-ileal intussusception in adults.Int J Surg Case Rep. 2017 Nov 21;41:469-472. doi: 10.1016/j.ijscr.2017.11.033. eCollection 2017. Int J Surg Case Rep. 2017. PMID: 29546019 Free PMC article.
-
Angiogenesis, Lymphangiogenesis, and the Immune Response in South African Preeclamptic Women Receiving HAART.Int J Mol Sci. 2019 Jul 30;20(15):3728. doi: 10.3390/ijms20153728. Int J Mol Sci. 2019. PMID: 31366152 Free PMC article. Review.
-
Update on the biology and treatment of lymphedema.Curr Treat Options Cardiovasc Med. 2012 Apr;14(2):184-92. doi: 10.1007/s11936-012-0170-0. Curr Treat Options Cardiovasc Med. 2012. PMID: 22382848
References
Publication types
MeSH terms
Substances
LinkOut - more resources
Full Text Sources