The crystal structure of yeast CCT reveals intrinsic asymmetry of eukaryotic cytosolic chaperonins
- PMID: 21701561
- PMCID: PMC3160183
- DOI: 10.1038/emboj.2011.208
The crystal structure of yeast CCT reveals intrinsic asymmetry of eukaryotic cytosolic chaperonins
Abstract
The cytosolic chaperonin CCT is a 1-MDa protein-folding machine essential for eukaryotic life. The CCT interactome shows involvement in folding and assembly of a small range of proteins linked to essential cellular processes such as cytoskeleton assembly and cell-cycle regulation. CCT has a classic chaperonin architecture, with two heterogeneous 8-membered rings stacked back-to-back, enclosing a folding cavity. However, the mechanism by which CCT assists folding is distinct from other chaperonins, with no hydrophobic wall lining a potential Anfinsen cage, and a sequential rather than concerted ATP hydrolysis mechanism. We have solved the crystal structure of yeast CCT in complex with actin at 3.8 Å resolution, revealing the subunit organisation and the location of discrete patches of co-evolving 'signature residues' that mediate specific interactions between CCT and its substrates. The intrinsic asymmetry is revealed by the structural individuality of the CCT subunits, which display unique configurations, substrate binding properties, ATP-binding heterogeneity and subunit-subunit interactions. The location of the evolutionarily conserved N-terminus of Cct5 on the outside of the barrel, confirmed by mutational studies, is unique to eukaryotic cytosolic chaperonins.
Conflict of interest statement
The authors declare that they have no conflict of interest.
Figures
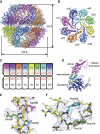
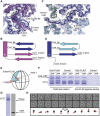
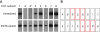
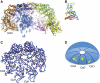
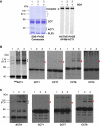
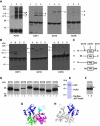
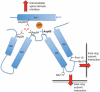
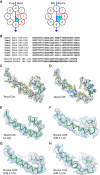
Similar articles
-
Molecular determinants of the ATP hydrolysis asymmetry of the CCT chaperonin complex.Proteins. 2014 May;82(5):703-7. doi: 10.1002/prot.24510. Epub 2014 Feb 12. Proteins. 2014. PMID: 24420718
-
Human CCT4 and CCT5 chaperonin subunits expressed in Escherichia coli form biologically active homo-oligomers.J Biol Chem. 2013 Jun 14;288(24):17734-44. doi: 10.1074/jbc.M112.443929. Epub 2013 Apr 23. J Biol Chem. 2013. PMID: 23612981 Free PMC article.
-
The crystal structures of the eukaryotic chaperonin CCT reveal its functional partitioning.Structure. 2013 Apr 2;21(4):540-9. doi: 10.1016/j.str.2013.01.017. Epub 2013 Mar 7. Structure. 2013. PMID: 23478063 Free PMC article.
-
Structure and function of a protein folding machine: the eukaryotic cytosolic chaperonin CCT.FEBS Lett. 2002 Oct 2;529(1):11-6. doi: 10.1016/s0014-5793(02)03180-0. FEBS Lett. 2002. PMID: 12354605 Review.
-
Mechanistic insights into protein folding by the eukaryotic chaperonin complex CCT.Biochem Soc Trans. 2022 Oct 31;50(5):1403-1414. doi: 10.1042/BST20220591. Biochem Soc Trans. 2022. PMID: 36196890 Free PMC article. Review.
Cited by
-
Kdm3a lysine demethylase is an Hsp90 client required for cytoskeletal rearrangements during spermatogenesis.Mol Biol Cell. 2014 Apr;25(8):1216-33. doi: 10.1091/mbc.E13-08-0471. Epub 2014 Feb 19. Mol Biol Cell. 2014. PMID: 24554764 Free PMC article.
-
Identification of an allosteric network that influences assembly and function of group II chaperonins.Nat Struct Mol Biol. 2017 Sep 7;24(9):683-684. doi: 10.1038/nsmb.3459. Nat Struct Mol Biol. 2017. PMID: 28880864 No abstract available.
-
Weak intra-ring allosteric communications of the archaeal chaperonin thermosome revealed by normal mode analysis.Biophys J. 2012 Sep 19;103(6):1285-95. doi: 10.1016/j.bpj.2012.07.049. Biophys J. 2012. PMID: 22995501 Free PMC article.
-
Mechanism of nucleotide sensing in group II chaperonins.EMBO J. 2012 Feb 1;31(3):731-40. doi: 10.1038/emboj.2011.468. Epub 2011 Dec 23. EMBO J. 2012. PMID: 22193720 Free PMC article.
-
Symmetry-free cryo-EM structures of the chaperonin TRiC along its ATPase-driven conformational cycle.EMBO J. 2012 Feb 1;31(3):720-30. doi: 10.1038/emboj.2011.366. Epub 2011 Nov 1. EMBO J. 2012. PMID: 22045336 Free PMC article.
References
-
- Adams PD, Grosse-Kunstleve RW, Hung L-W, Ioerger TR, McCoy AJ, Moriarty NW, Read RJ, Sacchettini JC, Sauter NK, Terwilliger TC (2002) PHENIX: building new software for automated crystallographic structure determination. Acta Crystallogr D Biol Crystallogr 58: 1948–1954 - PubMed
-
- Altschuler GM, Dekker C, McCormack A, Morris EP, Klug DR, Willison KR (2009) A single amino acid residue is responsible for species-specific incompatibility between CCT and alpha-actin. FEBS Lett 583: 782–786 - PubMed
-
- Amit M, Weisberg SJ, Nadler-Holly M, McCormack EA, Feldmesser E, Kaganovich D, Willison KR, Horovitz A (2010) Equivalent mutations in the eight subunits of the chaperonin CCT produce dramatically different cellular and gene expression phenotypes. J Mol Biol 401: 532–543 - PubMed
-
- Cong Y, Baker ML, Jakana J, Woolford D, Miller EJ, Reissmann S, Kumar RN, Redding-Johanson AM, Batth TS, Mukhopadhyay A, Ludtke SJ, Frydman J, Chiu W (2010) 4.0-A resolution cryo-EM structure of the mammalian chaperonin TRiC/CCT reveals its unique subunit arrangement. Proc Natl Acad Sci USA 107: 4967–4972 - PMC - PubMed
Publication types
MeSH terms
Substances
Grants and funding
LinkOut - more resources
Full Text Sources
Molecular Biology Databases