Pannexin 3 functions as an ER Ca(2+) channel, hemichannel, and gap junction to promote osteoblast differentiation
- PMID: 21690309
- PMCID: PMC3216329
- DOI: 10.1083/jcb.201101050
Pannexin 3 functions as an ER Ca(2+) channel, hemichannel, and gap junction to promote osteoblast differentiation
Abstract
The pannexin proteins represent a new gap junction family. However, the cellular functions of pannexins remain largely unknown. Here, we demonstrate that pannexin 3 (Panx3) promotes differentiation of osteoblasts and ex vivo growth of metatarsals. Panx3 expression was induced during osteogenic differentiation of C2C12 cells and primary calvarial cells, and suppression of this endogenous expression inhibited differentiation. Panx3 functioned as a unique Ca(2+) channel in the endoplasmic reticulum (ER), which was activated by purinergic receptor/phosphoinositide 3-kinase (PI3K)/Akt signaling, followed by activation of calmodulin signaling for differentiation. Panx3 also formed hemichannels that allowed release of ATP into the extracellular space and activation of purinergic receptors with the subsequent activation of PI3K-Akt signaling. Panx3 also formed gap junctions and propagated Ca(2+) waves between cells. Blocking the Panx3 Ca(2+) channel and gap junction activities inhibited osteoblast differentiation. Thus, Panx3 appears to be a new regulator that promotes osteoblast differentiation by functioning as an ER Ca(2+) channel and a hemichannel, and by forming gap junctions.
Figures
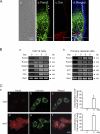
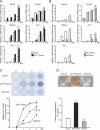
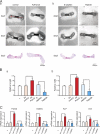
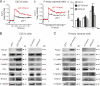
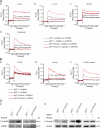
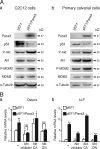
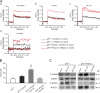
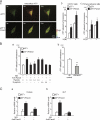
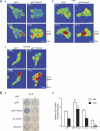
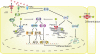
Similar articles
-
Pannexin 3 ER Ca2+ channel gating is regulated by phosphorylation at the Serine 68 residue in osteoblast differentiation.Sci Rep. 2019 Dec 10;9(1):18759. doi: 10.1038/s41598-019-55371-9. Sci Rep. 2019. PMID: 31822768 Free PMC article.
-
The Role of Pannexin 3 in Bone Biology.J Dent Res. 2017 Apr;96(4):372-379. doi: 10.1177/0022034516678203. Epub 2016 Nov 13. J Dent Res. 2017. PMID: 27837015 Free PMC article. Review.
-
Pannexin 3 inhibits proliferation of osteoprogenitor cells by regulating Wnt and p21 signaling.J Biol Chem. 2014 Jan 31;289(5):2839-51. doi: 10.1074/jbc.M113.523241. Epub 2013 Dec 12. J Biol Chem. 2014. PMID: 24338011 Free PMC article.
-
Pannexin-3 Deficiency Delays Skin Wound Healing in Mice due to Defects in Channel Functionality.J Invest Dermatol. 2019 Apr;139(4):909-918. doi: 10.1016/j.jid.2018.08.033. Epub 2018 Oct 30. J Invest Dermatol. 2019. PMID: 30389492
-
Pannexin 3 channels in health and disease.Purinergic Signal. 2021 Dec;17(4):577-589. doi: 10.1007/s11302-021-09805-7. Epub 2021 Jul 12. Purinergic Signal. 2021. PMID: 34250568 Free PMC article. Review.
Cited by
-
Manipulating connexin communication channels: use of peptidomimetics and the translational outputs.J Membr Biol. 2012 Aug;245(8):437-49. doi: 10.1007/s00232-012-9488-5. Epub 2012 Aug 11. J Membr Biol. 2012. PMID: 22886208 Free PMC article. Review.
-
Mechanisms of Pannexin 1 (PANX1) Channel Mechanosensitivity and Its Pathological Roles.Int J Mol Sci. 2022 Jan 28;23(3):1523. doi: 10.3390/ijms23031523. Int J Mol Sci. 2022. PMID: 35163442 Free PMC article. Review.
-
Differentiating connexin hemichannels and pannexin channels in cellular ATP release.FEBS Lett. 2014 Apr 17;588(8):1379-88. doi: 10.1016/j.febslet.2014.02.004. Epub 2014 Feb 15. FEBS Lett. 2014. PMID: 24548565 Free PMC article. Review.
-
Piezo1/2 mediate mechanotransduction essential for bone formation through concerted activation of NFAT-YAP1-ß-catenin.Elife. 2020 Mar 18;9:e52779. doi: 10.7554/eLife.52779. Elife. 2020. PMID: 32186512 Free PMC article.
-
Pannexin 1 channels: new actors in the regulation of catecholamine release from adrenal chromaffin cells.Front Cell Neurosci. 2014 Sep 4;8:270. doi: 10.3389/fncel.2014.00270. eCollection 2014. Front Cell Neurosci. 2014. PMID: 25237296 Free PMC article.
References
-
- Barbe M.T., Monyer H., Bruzzone R. 2006. Cell-cell communication beyond connexins: the pannexin channels. Physiology (Bethesda). 21:103–114 - PubMed
Publication types
MeSH terms
Substances
Grants and funding
LinkOut - more resources
Full Text Sources
Molecular Biology Databases
Miscellaneous