Molecular basis of positive allosteric modulation of GluN2B NMDA receptors by polyamines
- PMID: 21685875
- PMCID: PMC3160180
- DOI: 10.1038/emboj.2011.203
Molecular basis of positive allosteric modulation of GluN2B NMDA receptors by polyamines
Abstract
NMDA receptors (NMDARs) form glutamate-gated ion channels that have central roles in neuronal communication and plasticity throughout the brain. Dysfunctions of NMDARs are involved in several central nervous system disorders, including stroke, chronic pain and schizophrenia. One hallmark of NMDARs is that their activity can be allosterically regulated by a variety of extracellular small ligands. While much has been learned recently regarding allosteric inhibition of NMDARs, the structural determinants underlying positive allosteric modulation of these receptors remain poorly defined. Here, we show that polyamines, naturally occurring polycations that selectively enhance NMDARs containing the GluN2B subunit, bind at a dimer interface between GluN1 and GluN2B subunit N-terminal domains (NTDs). Polyamines act by shielding negative charges present on GluN1 and GluN2B NTD lower lobes, allowing their close apposition, an effect that in turn prevents NTD clamshell closure. Our work reveals the mechanistic basis for positive allosteric modulation of NMDARs. It provides the first example of an intersubunit binding site in this class of receptors, a discovery that holds promise for future drug interventions.
Conflict of interest statement
The authors declare that they have no conflict of interest.
Figures
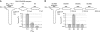
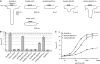
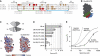
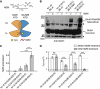
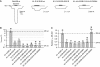
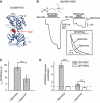
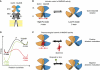
Similar articles
-
Subunit arrangement and phenylethanolamine binding in GluN1/GluN2B NMDA receptors.Nature. 2011 Jun 15;475(7355):249-53. doi: 10.1038/nature10180. Nature. 2011. PMID: 21677647 Free PMC article.
-
Allosteric signaling and dynamics of the clamshell-like NMDA receptor GluN1 N-terminal domain.Nat Struct Mol Biol. 2013 Apr;20(4):477-85. doi: 10.1038/nsmb.2522. Epub 2013 Mar 3. Nat Struct Mol Biol. 2013. PMID: 23454977
-
A Novel Binding Mode Reveals Two Distinct Classes of NMDA Receptor GluN2B-selective Antagonists.Mol Pharmacol. 2016 May;89(5):541-51. doi: 10.1124/mol.115.103036. Epub 2016 Feb 24. Mol Pharmacol. 2016. PMID: 26912815 Free PMC article.
-
Allosteric modulators of NR2B-containing NMDA receptors: molecular mechanisms and therapeutic potential.Br J Pharmacol. 2009 Aug;157(8):1301-17. doi: 10.1111/j.1476-5381.2009.00304.x. Epub 2009 Jul 8. Br J Pharmacol. 2009. PMID: 19594762 Free PMC article. Review.
-
Molecular basis of NMDA receptor functional diversity.Eur J Neurosci. 2011 Apr;33(8):1351-65. doi: 10.1111/j.1460-9568.2011.07628.x. Epub 2011 Mar 14. Eur J Neurosci. 2011. PMID: 21395862 Review.
Cited by
-
The Developmental Shift of NMDA Receptor Composition Proceeds Independently of GluN2 Subunit-Specific GluN2 C-Terminal Sequences.Cell Rep. 2018 Oct 23;25(4):841-851.e4. doi: 10.1016/j.celrep.2018.09.089. Cell Rep. 2018. PMID: 30355491 Free PMC article.
-
Positive surface charge of GluN1 N-terminus mediates the direct interaction with EphB2 and NMDAR mobility.Nat Commun. 2020 Jan 29;11(1):570. doi: 10.1038/s41467-020-14345-6. Nat Commun. 2020. PMID: 31996679 Free PMC article.
-
Subtype-dependent N-methyl-D-aspartate receptor amino-terminal domain conformations and modulation by spermine.J Biol Chem. 2015 May 15;290(20):12812-20. doi: 10.1074/jbc.M115.649723. Epub 2015 Mar 31. J Biol Chem. 2015. PMID: 25829490 Free PMC article.
-
The multifaceted subunit interfaces of ionotropic glutamate receptors.J Physiol. 2015 Jan 1;593(1):73-81. doi: 10.1113/jphysiol.2014.273409. Epub 2014 Jul 10. J Physiol. 2015. PMID: 25556789 Free PMC article. Review.
-
Targeting NMDA Receptors at the Neurovascular Unit: Past and Future Treatments for Central Nervous System Diseases.Int J Mol Sci. 2022 Sep 7;23(18):10336. doi: 10.3390/ijms231810336. Int J Mol Sci. 2022. PMID: 36142247 Free PMC article. Review.
References
-
- Armstrong N, Jasti J, Beich-Frandsen M, Gouaux E (2006) Measurement of conformational changes accompanying desensitization in an ionotropic glutamate receptor. Cell 127: 85–97 - PubMed
-
- Bertrand D, Gopalakrishnan M (2007) Allosteric modulation of nicotinic acetylcholine receptors. Biochem Pharmacol 74: 1155–1163 - PubMed
-
- Chesler M, Kaila K (1992) Modulation of pH by neuronal activity. Trends Neurosci 15: 396–402 - PubMed
-
- Choi YB, Lipton SA (1999) Identification and mechanism of action of two histidine residues underlying high-affinity Zn2+ inhibition of the NMDA receptor. Neuron 23: 171–180 - PubMed
Publication types
MeSH terms
Substances
LinkOut - more resources
Full Text Sources
Other Literature Sources
Molecular Biology Databases