Tumor-initiating stem cells of squamous cell carcinomas and their control by TGF-β and integrin/focal adhesion kinase (FAK) signaling
- PMID: 21670270
- PMCID: PMC3127891
- DOI: 10.1073/pnas.1107807108
Tumor-initiating stem cells of squamous cell carcinomas and their control by TGF-β and integrin/focal adhesion kinase (FAK) signaling
Abstract
Cancer stem cells (CSCs) sustain tumor growth through their ability to self-renew and to generate differentiated progeny. These functions endow CSCs with the potential to initiate secondary tumors bearing characteristics similar to those of the parent. Recently the hair follicle stem cell marker CD34 was used to purify a CSC-like cell population from early skin tumors arising from treatment with 7,12-dimethylbenz[α]anthracene/12-o-tetradecanoylphorbol-13-acetate, which typically generates benign papillomas that occasionally progress to squamous cell carcinomas (SCCs). In the present study, we identify and characterize CSCs purified from malignant SCCs. We show that SCCs contain two highly tumorigenic CSC populations that differ in CD34 levels but are enriched for integrins and coexist at the SCC-stroma interface. Intriguingly, whether CD34(lo) or CD34(hi), α6(hi)β1(hi) populations can initiate secondary tumors by serial limit-dilution transplantation assays, but α6(lo)β1(lo) populations cannot. Moreover, secondary tumors generated from a single CSC of either subtype contain both CD34(lo) and CD34(hi) α6(hi)β1(hi)CSCs, indicating their nonhierarchical organization. Genomic profiling and hierarchical cluster analysis show that these two CSC subtypes share a molecular signature distinct from either the CD34(-) epidermal or the CD34(hi) hair follicle stem cell signature. Although closely related, α6(hi)β1(hi)CD34(lo) and α6(hi)β1(hi)CD34(hi) CSCs differ in cell-cycle gene expression and proliferation characteristics. Indeed, proliferation and expansion of α6(hi)β1(hi)CD34(hi) CSCs is sensitive to whether they can initiate a TGF-β receptor II-mediated response to counterbalance elevated focal adhesion kinase-mediated integrin signaling within the tumor. Overall, the coexistence and interconvertibility of CSCs with differing sensitivities to their microenvironment pose challenges and opportunities for SCC cancer therapies.
Conflict of interest statement
The authors declare no conflict of interest.
Figures
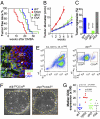
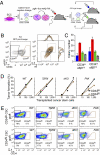
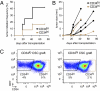
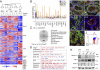
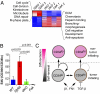
Similar articles
-
Cancer stem-like cells enriched with CD29 and CD44 markers exhibit molecular characteristics with epithelial-mesenchymal transition in squamous cell carcinoma.Arch Dermatol Res. 2013 Jan;305(1):35-47. doi: 10.1007/s00403-012-1260-2. Epub 2012 Jun 28. Arch Dermatol Res. 2013. PMID: 22740085
-
Cancer stem cells and their niche in the progression of squamous cell carcinoma.Cancer Sci. 2020 Nov;111(11):3985-3992. doi: 10.1111/cas.14639. Epub 2020 Sep 18. Cancer Sci. 2020. PMID: 32888236 Free PMC article. Review.
-
Therapeutic targeting of the focal adhesion complex prevents oncogenic TGF-beta signaling and metastasis.Breast Cancer Res. 2009;11(5):R68. doi: 10.1186/bcr2360. Breast Cancer Res. 2009. PMID: 19740433 Free PMC article.
-
SOX2 controls tumour initiation and cancer stem-cell functions in squamous-cell carcinoma.Nature. 2014 Jul 10;511(7508):246-50. doi: 10.1038/nature13305. Epub 2014 Jun 8. Nature. 2014. PMID: 24909994
-
Cancer Stem Cells in Squamous Cell Carcinoma.J Invest Dermatol. 2017 Jan;137(1):31-37. doi: 10.1016/j.jid.2016.07.033. Epub 2016 Nov 24. J Invest Dermatol. 2017. PMID: 27638386 Free PMC article. Review.
Cited by
-
Serial orthotopic transplantation of epithelial tumors in single-cell suspension.Methods Mol Biol. 2013;1035:231-45. doi: 10.1007/978-1-62703-508-8_20. Methods Mol Biol. 2013. PMID: 23959996 Free PMC article.
-
WNT ligands control initiation and progression of human papillomavirus-driven squamous cell carcinoma.Oncogene. 2018 Jul;37(27):3753-3762. doi: 10.1038/s41388-018-0244-x. Epub 2018 Apr 17. Oncogene. 2018. PMID: 29662191 Free PMC article.
-
KRAB-ZFPs and cancer stem cells identity.Genes Dis. 2022 Apr 9;10(5):1820-1832. doi: 10.1016/j.gendis.2022.03.013. eCollection 2023 Sep. Genes Dis. 2022. PMID: 37492743 Free PMC article. Review.
-
Prevention of tumor risk associated with the reprogramming of human pluripotent stem cells.J Exp Clin Cancer Res. 2020 Jun 3;39(1):100. doi: 10.1186/s13046-020-01584-0. J Exp Clin Cancer Res. 2020. PMID: 32493501 Free PMC article. Review.
-
3D genome mapping identifies subgroup-specific chromosome conformations and tumor-dependency genes in ependymoma.Nat Commun. 2023 Apr 21;14(1):2300. doi: 10.1038/s41467-023-38044-0. Nat Commun. 2023. PMID: 37085539 Free PMC article.
References
-
- Hanahan D, Weinberg RA. The hallmarks of cancer. Cell. 2000;100:57–70. - PubMed
-
- He S, Nakada D, Morrison SJ. Mechanisms of stem cell self-renewal. Annu Rev Cell Dev Biol. 2009;25:377–406. - PubMed
-
- Clarke MF, et al. Cancer stem cells—perspectives on current status and future directions: AACR Workshop on cancer stem cells. Cancer Res. 2006;66:9339–9344. - PubMed
-
- Clevers H. The cancer stem cell: Premises, promises and challenges. Nat Med. 2011;17:313–319. - PubMed
Publication types
MeSH terms
Substances
Associated data
- Actions
Grants and funding
LinkOut - more resources
Full Text Sources
Other Literature Sources
Medical
Molecular Biology Databases
Research Materials
Miscellaneous