The JAK2/STAT3 signaling pathway is required for growth of CD44⁺CD24⁻ stem cell-like breast cancer cells in human tumors
- PMID: 21633165
- PMCID: PMC3223826
- DOI: 10.1172/JCI44745
The JAK2/STAT3 signaling pathway is required for growth of CD44⁺CD24⁻ stem cell-like breast cancer cells in human tumors
Abstract
Intratumor heterogeneity is a major clinical problem because tumor cell subtypes display variable sensitivity to therapeutics and may play different roles in progression. We previously characterized 2 cell populations in human breast tumors with distinct properties: CD44+CD24- cells that have stem cell-like characteristics, and CD44-CD24+ cells that resemble more differentiated breast cancer cells. Here we identified 15 genes required for cell growth or proliferation in CD44+CD24- human breast cancer cells in a large-scale loss-of-function screen and found that inhibition of several of these (IL6, PTGIS, HAS1, CXCL3, and PFKFB3) reduced Stat3 activation. We found that the IL-6/JAK2/Stat3 pathway was preferentially active in CD44+CD24- breast cancer cells compared with other tumor cell types, and inhibition of JAK2 decreased their number and blocked growth of xenografts. Our results highlight the differences between distinct breast cancer cell types and identify targets such as JAK2 and Stat3 that may lead to more specific and effective breast cancer therapies.
Figures
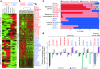
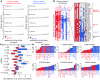
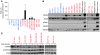
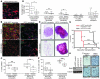
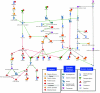
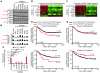
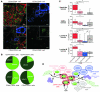
Similar articles
-
Evaluation of STAT3 signaling in ALDH+ and ALDH+/CD44+/CD24- subpopulations of breast cancer cells.PLoS One. 2013 Dec 23;8(12):e82821. doi: 10.1371/journal.pone.0082821. eCollection 2013. PLoS One. 2013. PMID: 24376586 Free PMC article.
-
Loss of miR-6844 alters stemness/self-renewal and cancer hallmark(s) markers through CD44-JAK2-STAT3 signaling axis in breast cancer stem-like cells.J Cell Biochem. 2023 Aug;124(8):1186-1202. doi: 10.1002/jcb.30441. Epub 2023 Jul 12. J Cell Biochem. 2023. PMID: 37436061
-
P16 promotes the growth and mobility potential of breast cancer both in vitro and in vivo: the key role of the activation of IL-6/JAK2/STAT3 signaling.Mol Cell Biochem. 2018 Sep;446(1-2):137-148. doi: 10.1007/s11010-018-3281-4. Epub 2018 Jan 31. Mol Cell Biochem. 2018. PMID: 29388151
-
Breast cancer stem cells and intrinsic subtypes: controversies rage on.Curr Stem Cell Res Ther. 2009 Jan;4(1):50-60. doi: 10.2174/157488809787169110. Curr Stem Cell Res Ther. 2009. PMID: 19149630 Review.
-
Timing somatic events in the evolution of cancer.Genome Biol. 2018 Jul 24;19(1):95. doi: 10.1186/s13059-018-1476-3. Genome Biol. 2018. PMID: 30041675 Free PMC article. Review.
Cited by
-
The Role of Salivary Vascular Endothelial Growth Factor A, Cytokines, and Amino Acids in Immunomodulation and Angiogenesis in Breast Cancer.Biomedicines. 2024 Jun 14;12(6):1329. doi: 10.3390/biomedicines12061329. Biomedicines. 2024. PMID: 38927536 Free PMC article.
-
WNT10B/β-catenin signalling induces HMGA2 and proliferation in metastatic triple-negative breast cancer.EMBO Mol Med. 2013 Feb;5(2):264-79. doi: 10.1002/emmm.201201320. Epub 2013 Jan 11. EMBO Mol Med. 2013. PMID: 23307470 Free PMC article.
-
HN1 as a diagnostic and prognostic biomarker for liver cancer.Biosci Rep. 2020 Jul 31;40(7):BSR20200316. doi: 10.1042/BSR20200316. Biosci Rep. 2020. PMID: 32700728 Free PMC article.
-
Curcumin and epigallocatechin gallate inhibit the cancer stem cell phenotype via down-regulation of STAT3-NFκB signaling.Anticancer Res. 2015 Jan;35(1):39-46. Anticancer Res. 2015. PMID: 25550533 Free PMC article.
-
P2Y2R has a significant correlation with Notch-4 in patients with breast cancer.Oncol Lett. 2020 Jul;20(1):647-654. doi: 10.3892/ol.2020.11630. Epub 2020 May 14. Oncol Lett. 2020. PMID: 32565989 Free PMC article.
References
Publication types
MeSH terms
Substances
Grants and funding
LinkOut - more resources
Full Text Sources
Other Literature Sources
Medical
Molecular Biology Databases
Miscellaneous