Spinal vascular endothelial growth factor induces phrenic motor facilitation via extracellular signal-regulated kinase and Akt signaling
- PMID: 21613481
- PMCID: PMC3172810
- DOI: 10.1523/JNEUROSCI.0239-11.2011
Spinal vascular endothelial growth factor induces phrenic motor facilitation via extracellular signal-regulated kinase and Akt signaling
Abstract
Although vascular endothelial growth factor (VEGFA-165) is primarily known for its role in angiogenesis, it also plays important neurotrophic and neuroprotective roles for spinal motor neurons. VEGFA-165 signals by activating its receptor tyrosine kinase VEGF receptor-2 (VEGFR-2). Because another growth/trophic factor that signals via a receptor tyrosine kinase (brain derived neurotrophic factor) elicits a long-lasting facilitation of respiratory motor activity in the phrenic nerve, we tested the hypothesis that VEGFA-165 elicits similar phrenic motor facilitation (pMF). Using immunohistochemistry and retrograde labeling techniques, we demonstrate that VEGFA-165 and VEGFR-2 are expressed in identified phrenic motor neurons. Furthermore, intrathecal VEGFA-165 administration at C4 elicits long-lasting pMF; intraspinal VEGFA-165 increased integrated phrenic nerve burst amplitude for at least 90 min after injection (53.1 ± 5.0% at 90 min; p < 0.001). Intrathecal VEGFA-165 increased phosphorylation (and presumed activation) of signaling molecules downstream from VEGFR-2 within the phrenic motor nucleus, including ERK (1.53 ± 0.13 vs 1.0 ± 0.05 arbitrary units in control rats; p < 0.05) and Akt (2.16 ± 0.41 vs 1.0 ± 0.41 arbitrary units in control rats; p < 0.05). VEGF-induced pMF was attenuated by the MEK/ERK inhibitor U0126 [1,4-diamino-2,3-dicyano-1,4-bis(o-aminophenylmercapto)butadiene] and was abolished by the phosphotidinositol 3 kinase/Akt inhibitor LY294002 [2-(4-morpholinyl)-8-phenyl-1(4H)-benzopyran-4-one hydrochloride], demonstrating that ERK mitogen-activated protein kinases and Akt are both required for full expression of VEGF-induced pMF. This is the first report that VEGFA-165 elicits plasticity in any motor system. Furthermore, because VEGFA-165 expression is hypoxia sensitive, it may play a role in respiratory plasticity after prolonged exposures to low oxygen.
Figures
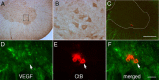
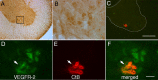
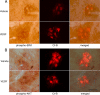
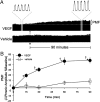
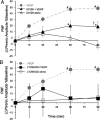
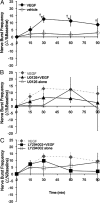
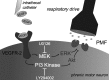
Similar articles
-
Cervical spinal erythropoietin induces phrenic motor facilitation via extracellular signal-regulated protein kinase and Akt signaling.J Neurosci. 2012 Apr 25;32(17):5973-83. doi: 10.1523/JNEUROSCI.3873-11.2012. J Neurosci. 2012. PMID: 22539857 Free PMC article.
-
Spinal BDNF-induced phrenic motor facilitation requires PKCθ activity.J Neurophysiol. 2017 Nov 1;118(5):2755-2762. doi: 10.1152/jn.00945.2016. Epub 2017 Aug 30. J Neurophysiol. 2017. PMID: 28855298 Free PMC article.
-
BDNF-induced phrenic motor facilitation shifts from PKCθ to ERK dependence with mild systemic inflammation.J Neurophysiol. 2023 Feb 1;129(2):455-464. doi: 10.1152/jn.00345.2022. Epub 2023 Jan 25. J Neurophysiol. 2023. PMID: 36695529 Free PMC article.
-
Circulatory control of phrenic motor plasticity.Respir Physiol Neurobiol. 2019 Jul;265:19-23. doi: 10.1016/j.resp.2019.01.004. Epub 2019 Jan 11. Respir Physiol Neurobiol. 2019. PMID: 30639504 Free PMC article. Review.
-
NADPH oxidase activity is necessary for acute intermittent hypoxia-induced phrenic long-term facilitation.J Physiol. 2009 May 1;587(Pt 9):1931-42. doi: 10.1113/jphysiol.2008.165597. Epub 2009 Feb 23. J Physiol. 2009. PMID: 19237427 Free PMC article. Review.
Cited by
-
Spinal protein phosphatase 1 constrains respiratory plasticity after sustained hypoxia.J Appl Physiol (1985). 2018 Nov 1;125(5):1440-1446. doi: 10.1152/japplphysiol.00641.2018. Epub 2018 Aug 30. J Appl Physiol (1985). 2018. PMID: 30161006 Free PMC article.
-
Emerging roles for semaphorins and VEGFs in synaptogenesis and synaptic plasticity.Cell Adh Migr. 2012 Nov-Dec;6(6):541-6. doi: 10.4161/cam.22408. Epub 2012 Oct 17. Cell Adh Migr. 2012. PMID: 23076132 Free PMC article. Review.
-
Impact of inflammation on developing respiratory control networks: rhythm generation, chemoreception and plasticity.Respir Physiol Neurobiol. 2020 Mar;274:103357. doi: 10.1016/j.resp.2019.103357. Epub 2019 Dec 30. Respir Physiol Neurobiol. 2020. PMID: 31899353 Free PMC article. Review.
-
Repeated intravenous doxapram induces phrenic motor facilitation.Exp Neurol. 2013 Dec;250:108-15. doi: 10.1016/j.expneurol.2013.08.016. Epub 2013 Sep 4. Exp Neurol. 2013. PMID: 24013015 Free PMC article.
-
Spinal activation of protein kinase C elicits phrenic motor facilitation.Respir Physiol Neurobiol. 2018 Oct;256:36-42. doi: 10.1016/j.resp.2017.10.007. Epub 2017 Nov 2. Respir Physiol Neurobiol. 2018. PMID: 29081358 Free PMC article.
References
-
- Acker T, Beck H, Plate KH. Cell type specific expression of vascular endothelial growth factor and angiopoietin-1 and -2 suggests an important role of astrocytes in cerebellar vascularization. Mech Dev. 2001;108:45–57. - PubMed
-
- Atkins CM, Selcher JC, Petraitis JJ, Trzaskos JM, Sweatt JD. The MAPK cascade is required for mammalian associative learning. Nat Neurosci. 1998;1:602–609. - PubMed
-
- Bach KB, Mitchell GS. Hypoxia-induced long-term facilitation of respiratory activity is serotonin dependent. Respir Physiol. 1996;104:251–260. - PubMed
-
- Baker-Herman TL, Fuller DD, Bavis RW, Zabka AG, Golder FJ, Doperalski NJ, Johnson RA, Watters JJ, Mitchell GS. BDNF is necessary and sufficient for spinal respiratory plasticity following intermittent hypoxia. Nat Neurosci. 2004;7:48–55. - PubMed
Publication types
MeSH terms
Substances
Grants and funding
LinkOut - more resources
Full Text Sources
Other Literature Sources
Miscellaneous